
rexresearch.com
Jian Ping Gong, et al.
Fiber-Reinforced Soft Composite
http://www.sciencealert.com/scientists-invent-a-hydrogel-fabric-that-s-five-times-stronger-than-steel
Scientists Have Invented a Hydrogel Fabric
That's 5 Times Stronger Than Steel
But you can still bend and stretch it.
by DAVID NIELD
Scientists have created a new hydrogel material reinforced with
fibres that they say is up to five times harder to break than
carbon steel – but still easy to bend and stretch.
That combination of properties means the new fabric could be used
as the basis for artificial ligaments and tendons designed to help
the body heal – or in manufacturing or fashion where a very tough
but elastic material is needed.
Researchers from Hokkaido University in Japan developed the
fabric, called fibre-reinforced soft composite (or FRSC), by
combining hydrogels containing high levels of water with glass
fibre fabric....
The end result is a material that's 25 times tougher than glass
fibre fabric; 100 times tougher than hydrogels; and five times as
strong as carbon steel, in terms of the energy required to break
them...
https://www.oia.hokudai.ac.jp/blog/new-tougher-than-metal-fiber-reinforced-hydrogels/
New “tougher-than-metal” fiber-reinforced
hydrogels
A team of Hokkaido University scientists has succeeded in creating
“fiber-reinforced soft composites,” or tough hydrogels combined
with woven fiber fabric. These fabrics are highly flexible,
tougher than metals, and have a wide range of potential
applications.
Efforts are currently underway around the world to create
materials that are friendly to both society and the environment.
Among them are those that comprise different materials, which
exhibit the merits of each component.
Hokkaido University researchers, led by Professor Jian Ping Gong,
have focused on creating a reinforced material using hydrogels.
Though such a substance has potential as a structural biomaterial,
up until now no material reliable and strong enough for long-term
use has been produced. This study was conducted as a part of the
Cabinet Office’s Impulsing Paradigm Change through Disruptive
Technologies Program (ImPACT).
To address the problem, the team combined hydrogels containing
high levels of water with glass fiber fabric to create bendable,
yet tough materials, employing the same method used to produce
reinforced plastics. The team found that a combination of
polyampholyte (PA) gels, a type of hydrogel they developed
earlier, and glass fiber fabric with a single fiber measuring
around 10µm in diameter produced a strong, tensile material. The
procedure to make the material is simply to immerse the fabric in
PA precursor solutions for polymerization.
When used alone, the fiber-reinforced hydrogels developed by the
team are 25 times tougher than glass fiber fabric, and 100 times
tougher than hydrogels – in terms of the energy required to
destroy them. Combining these materials enables a synergistic
toughening. The team theorizes that toughness is increased by
dynamic ionic bonds between the fiber and hydrogels, and within
the hydrogels, as the fiber’s toughness increases in relation to
that of the hydrogels. Consequently, the newly developed hydrogels
are 5 times tougher compared to carbon steel.
“The fiber-reinforced hydrogels, with a 40 percent water level,
are environmentally friendly,” says Dr. Jian Ping Gong, “The
material has multiple potential applications because of its
reliability, durability and flexibility. For example, in addition
to fashion and manufacturing uses, it could be used as artificial
ligaments and tendons, which are subject to strong load-bearing
tensions.” The principles to create the toughness of the present
study can also be applied to other soft components, such as
rubber.
Fig2-Ionic bonds
Scanning Electron Microscopy (SEM) images of the fiber-reinforced
hydrogels. The polymer matrix (arrows) filled the interstitial
space in the fiber bundles and connected the neighboring fibers.
(Huang Y. et al., Advanced Functional Materials, January 16, 2017)
Contacts:
Professor Jiang Ping Gong
Graduate School of Life Science
Hokkaido University
Email: gong[at]sci.hokudai.ac.jp
Naoki Namba (Media Officer)
Global Relations Office
Institute for International Collaboration
Hokkaido University
Tel: +81-11-706-8034
Email: pr[at]oia.hokudai.ac.jp
http://onlinelibrary.wiley.com/doi/10.1002/adfm.201605350/abstract
DOI: 10.1002/adfm.201605350
Advanced Functional Materials, January 16, 2017.
Energy-Dissipative Matrices Enable
Synergistic Toughening in Fiber Reinforced Soft Composites
Yiwan Huang, et al.
Abstract
Tough hydrogels have shown strong potential as structural
biomaterials. These hydrogels alone, however, possess limited
mechanical properties (such as low modulus) when compared to some
load-bearing tissues, e.g., ligaments and tendons. Developing both
strong and tough soft materials is still a challenge. To overcome
this obstacle, a new material design strategy has been recently
introduced by combining tough hydrogels with woven fiber fabric to
create fiber reinforced soft composites (FRSCs). The new FRSCs
exhibit extremely high toughness and tensile properties, far
superior to those of the neat components, indicating a synergistic
effect. Here, focus is on understanding the role of energy
dissipation of the soft matrix in the synergistic toughening of
FRSCs. By selecting a range of soft matrix materials, from tough
hydrogels to weak hydrogels and even a commercially available
elastomer, the toughness of the matrix is determined to play a
critical role in achieving extremely tough FRSCs. This work
provides a good guide toward the universal design of soft
composites with extraordinary fracture resistance capacity.
http://pubs.rsc.org/en/content/articlehtml/2015/mh/c5mh00127g
DOI: 10.1039/C5MH00127G
(Communication) Mater. Horiz., 2015, 2, 584-591
Extremely tough composites from fabric
reinforced polyampholyte hydrogels
Daniel R. King, et al.
Ligaments are unique wet biological tissues with high tensile
modulus and fracture stress, combined with high bending
flexibility. Developing synthetic materials with these properties
is a significant challenge. Hydrogel composites made from high
stiffness fabrics is a strategy to develop such unique materials;
however, the ability to produce these materials has proven
difficult, since common hydrogels swell in water and interact
poorly with solid components, limiting the transfer of force from
the fabric to the hydrogel matrix. In this work, for the first
time, we successfully produce extraordinarily tough hydrogel
composites by strategically selecting a recently developed tough
hydrogel that de-swells in water. The new composites, consisting
of polyampholyte hydrogels and glass fiber woven fabrics, exhibit
extremely high effective toughness (250[thin space (1/6-em)]000 J
m−2), high tear strength (∼65 N mm−1), high tensile modulus (606
MPa), and low bending modulus (4.7 MPa). Even though these
composites are composed of water-containing, biocompatible
materials, their mechanical properties are comparable to high
toughness Kevlar/polyurethane blends and fiber-reinforced
polymers. Importantly, the mechanical properties of these
composites greatly outperform the properties of either individual
component. A mechanism is proposed based on established fabric
tearing theory, which will enable the development of a new
generation of mechanically robust composites based on fabrics.
These results will be important towards developing soft biological
prosthetics, and more generally for commercial applications such
as tear-resistant gloves and bulletproof vests.
WO2016027383
COMPOSITE COMPRISING FABRIC AND POLYAMPHOLYTE HYDROGEL AND
PREPARATION METHOD THEREOF
Inventor(s): GONG JIAN PING, et al.
A composite comprising a fabric and a polyampholyte hydrogel is
provided. In the composite, the polyampholyte hydrogel is a
hydrogel of a polymer bearing randomly dispersed cationic and
anionic repeat groups and at least a part of the fabric is coated
with the polyampholyte hydrogel. A method of preparation of the
composite comprises steps (a) to (c): (a) providing a monomer
mixture for preparation of a polyampholyte hydrogel; (b) immersing
a fabric in the monomer mixture solution; and (c) polymerizing
monomers in the monomer mixture solution to obtain a precursor of
the composite.
DESCRIPTION
Field of The Invention
This invention relates to a composite comprising a fabric and a
polyampholyte hydrogel and a method of preparation of the
composite.
Discussion of The Background
Anterior Cruciate Ligament (ACL) reconstruction surgeries are one
of the most common surgeries performed by orthopedic surgeons. To
avoid using autographs or allographs, synthetic materials have
been investigated as potential replacements.
Some materials which have been tested are Dacron, Marlex, Teflon,
Nylon, and Carbon Fiber. [NP-2] It is important that the materials
which are used can support the high load placed upon these
ligaments. Carbon Fiber has been especially of interest as a
replacement material because of its extremely high stiffness.
Initial results with neat carbon fiber replacements were
promising. [NP-3, 4] However problems were encountered, including
fracture, fraying and spreading of carbon in the body, irritation
and poor healing of the surrounding area, and accumulation of
carbon in regional lymph nodes. [NP- 1,2, 5]
NP-1 : K. Miller, J. E. Hsu, L. J. Soslowsky, in Comprehensive
Biomaterials, 2011, pp. 257-279.
NP-2: A. A. Amis, S. A. Kempson, J. R. Campbell, J. H. Miller,
Journal of Bone and Joint Surgery 1988, 70-B, 628-634.
NP-3: D. H. R. Jenkins, B. McKibbin, Journal of Bone and Joint
Surgery 1980, 62, 497-499.
NP-4: D. H. R. Jenkins, Journal of Bone and Joint Surgery 1978,
60-B, 520-522.
NP-5: S. Ramakrishna, J. Mayer, Composites Science and ... 2001,
61, 1189-1224.
Some researchers have also attempted to use hydrogels as ligament
replacements, but they lack the mechanical properties to support
high loads. There is some mention in the patent literature of
hydrogel and fabrics as prosthetics. [P-l, 2, 3, 4]
P-l : US Patent No. 6,629,997.
P-2: US Patent No. 6,187,043.
P-3: US Patent No. 6,027,744.
P-4: US Patent No. 4,919,667.
However the brittleness of traditional hydrogels may have
prevented these methods from entering the market.
In addition to the application in ACL reconstruction surgeries,
the applications listed below are those in which high toughness is
desired, such as skin grafts, flexible tear resistant clothing,
bandaging, bullet-proofing, etc.
In bandages, there is very little in the literature about fabric /
hydrogel bandages, however there is some mention in the patent
literature. In these systems fabric are used as support, and the
hydrogel is used for wound closure, as they are generally
non-toxic, consist mostly of water, and can promote wound healing.
[P-5 to P-8]
P-5 : Absorptive wound dressing for wound healing promotion -
5,695,777 ;
P-6: Dressing material based on a hydrogel, and a process for its
production -4,552,138 ;
P-7: Reinforced, laminated, impregnated, and composite-like
materials as crosslinked polyvinyl alcohol hydrogel structures -
6,855,743;
P-8: Hydrogel Laminate, Bandages, and composites and methods for
forming the same - 5,674,346
However, within these patents there is no obvious mention of
utilizing these composite materials to make extremely tough or
tear resistant bandages.
Fabric composites have been looked at and utilized before as high
strength and toughness materials. Some of these are for ballistics
and blast resistance.
P-9: Reinforced film for blast resistance protection - 8,039,102;
P-10: Impact resistive composite materials and methods for making
same - 7,608,322;
P-l l : Body armor with improved knife-stab resistance formed from
flexible composites - 7,288,493;
P-12: Ballistic-resistant article and process for making the same
- 6,268,301;
P-13: Ballistic fabric laminates - 6,846,758;
P-14: Armor panel - 5,789,327;
P-15: Method for improving the energy absorption of a high
tenacity fabric during a ballistic event - 5,595,809;
P-16: Lightweight armor and ballistic projectile defense apparatus
- 8,375,839).
There are materials used for airbag technologies in vehicles.
P-17: Silicone composition for coating substrates made of textile
material - 6,586,551 ;
P-18: Silicone coated textile fabrics - 6,354,620; etc.
Finally, some patents mention fabrics which exhibit high tear
strength.
P-19: Textile-reinforced composites with high tear strength -
7,549,303;
P-20: Method of making a cut and abrasion resistant laminate -
6,280,546;
P-21 : Elastic composite structure - 7,153,789;
P-22: Composite elastic material - 6,706,364;
P-23: Cut resistant yarn, fabric and gloves - 5,119,512;
P-24: Fiber reinforced thermosetting resin compositions with
coated fibers for improved toughness - 4,737,527;
P-25: Method of manufacture of silicone elastomer coated cloth -
4,478,895.
However in these examples, none of the composite materials utilize
hydrogels, or any type of elastic materials which contain water.
In many cases, the matrix material is thermosetting, which would
result in the composite lacking any flexibility.
Recently, new polyampholyte (PA) hydrogels produced by Sun et. al.
have shown the ability to maintain these features through a
macromolecular architecture that also features self-healing, and
one-pot synthesis abilities. [NP-6,7]
NP-6: T. L. Sun, T. Kurokawa, S. Kuroda, A. Bin Ihsan, T. Akasaki,
K. Sato, M. A. Haque, T. Nakajima, J. P. Gong, Nature materials
2013, 12, 1-6.
NP-7: A. Bin Ihsan, T. L. Sun, S. Kuroda, M. A. Haque, T.
Kurokawa, T.Nakajimac, J. P. Gong, J. Mater. Chem. B, 2013, 1 ,
4555-4562
Previous work from the Crosby Research Group has shown that
embedding soft elastomers with stiff fabrics can result in very
interesting composite materials. [NP-8 to NP-10] Double network
hydrogels devolved in the Gong Research Group have extremely high
toughness, while having moderate ultimate tensile strength and low
friction. [NP 11-14].
NP-8: M. D. Bartlett, A. B. Croll, D. R. King, B. M. Paret, D. J.
Irshick, A. J.Crosby, Advanced Materials 2012, 24, 1078-1083.
NP-9: S. A. Pendergraph, M. D. Bartlett, K. R. Carter, A. J.
Crosby, ACS Applied Materials & Interfaces 2012, 4, 6640-5.
NP-10: D. R. King, M. D. Bartlett, C. A. Gilman, D. J. Irschick,
A. J. Crosby, in prep 2013.
NP-11 : J. P. Gong, Y. Katsuyama, T. Kurokawa, Y. Osada, Advanced
Materials 2003, 15, 1155-1158. Revised 12/17/12 4
NP-12: J. P. Gong, T. Kurokawa, T. Narita, G. Kagata, Y. Osada, G
Nishimura, M. Kinjo, Journal of the American Chemical Society
2001, 123, 5582-5583.
NP-13: T. Tominaga, N. Takedomi, H. Biederman, H. Furukawa, Y.
Osada, J. P.Gong, Soft Matter 2008, 4, 1033.
NP-14: K. Yasuda, J. P. Gong, Y. Katsuyama, A. Nakayama, Y.
Tanabe, E. Kondo, M. Ueno, Y. Osada, Biomaterials 2005, 26,
4468-4475.
Utilizing a fabric and PA hydrogel composite could allow for high
loads (from the fiber) while the PA hydrogel could prevent wear
and rejection from the body. Incorporating these new hydrogels
into fabric will allow for new materials with unique properties
that are currently lacking in the literatures and patents.
Brief Description of The Drawings
The present invention will be described in the following text by
the exemplary, non-limiting embodiments shown in the figures,
wherein:
Figure 1 is a computer-aided drawing of one embodiment of the
composites of the present invention. Left: a side view; right: a
projection view.
Figure 2 is an image of testing setup for the tearing test.
Figure 3 is a tearing test result showing tear strength at
breakage on one embodiment of the composite of the present
invention. Comparison of a neat glass fiber fabric, a PA
hydrogel, a combination of the fabric and a SN hydrogel, the SN
hydrogel.
Figure 4 shows force vs. displacement curve for 20 mm wide
samples of the composite of the present invention, a neat glass
fiber fabric, a combination of the fabric and a SN hydrogel.
Figure 5 shows microscopy images of glass fiber fabric and
PA hydrogel between the fibers of the fabric of one embodiment
of the composite of the present invention.
Figure 6 is a microscopy image showing deformation in the
tearing region of the fabric (macro scale)
Figure 7 is a tensile stress-strain measurement result on
one embodiment of the composite of the present invention.
Figure 8 is a tensile stress-strain measurement result on a
neat glass fabric.
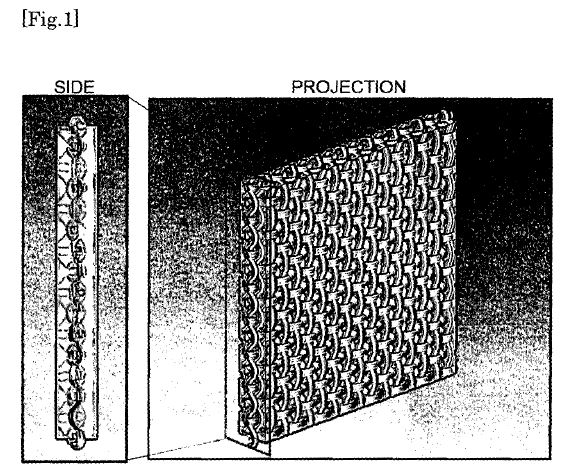
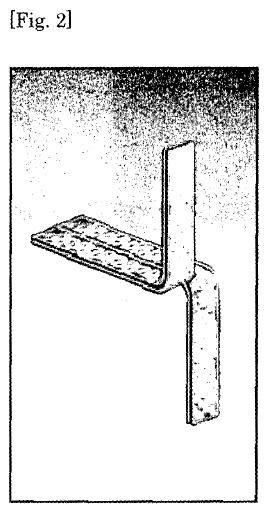
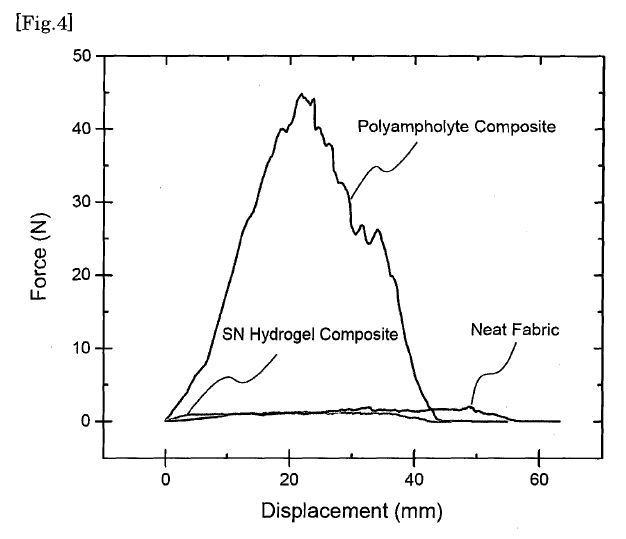
Descriptions of The Embodiments
The following preferred specific embodiments are, therefore, to be
construed as merely illustrative, and not limitative of the
remainder of the disclosure in any way whatsoever. In this regard,
no attempt is made to show structural details of the present
invention in more detail than is necessary for the fundamental
understanding of the present invention, the description taken with
the drawings making apparent to those skilled in the art how the
several forms of the present invention may be embodied in
practice.
This invention relates to a composite comprising a fabric and a PA
hydrogel which is relatively soft and viscoelastic. The fabric can
be a woven fabric or unwoven fabric and the unwoven fabric can be
a knitted fabric. A woven fabric is obtained by producing the
interlacing of warp fibers and weft fibers in weave styles, such
as plain, twill, or satin styles and so on. A woven fabric is
preferred because the integrity of the woven fabric is maintained
by the mechanical interlocking of the fibers, and their drape,
surface smoothness and stability can be controlled by the weave
styles based on the design requirements.
The fabric can be made from inorganic material fibers, organic
material fibers, biomaterial fibers or a mixture thereof.
Inorganic material fibers are composed of inorganic chemical
compounds, based on natural elements, which are resistant to high
temperatures and high mechanical strength. Examples of the
inorganic material fibers include glass fibers, carbon fibers,
ceramics fibers and metals fibers. These inorganic materials
fibers are commonly applied infields that require high performance
materials, such as building materials, protective clothing, parts
of airplanes, spaceships, and so on. Organic material fibers are
made from synthesized polymers or small molecules, which are
polymerized into long linear chains. Examples of the organic
material fibers include synthetic polymer fibers such as nylon
fibers, aramid fibers, polyalkylene fibers such as polyethylene
fibers, polypropylene fibers. These organic material fibers with
high mechanical performance and thermal stability are reinforced
in the polymer matrix, which are applied in fields such as the
automotive industry, railways, aerospace, and so on. Biomaterial
fibers are made from plants and animals, which are low cost, low
density, high toughness, renewable and biodegradable. Examples of
biomaterial fibers include cellulose fibers and cotton fibers.
These biomaterials fibers are widely reinforced with a polymer
matrix in the textile, and automotive industries. The fabric is
preferably comprised of long fibers. Woven and non-woven fabrics
that are commercially available can be used. The fabric can be in
a form of a sheet, a tape, a strip, a tube, a rod, and so on. The
preferred composite is produced by the fabric with hydrophilic
surface, such as glass fiber, and PA hydrogel.
In the composite of this invention, at least a part of the surface
of the fabric is coated with the PA hydrogel. The PA hydrogel can
be coated on either surface of the fabric or both surface of the
fabric partially or fully. The PA hydrogel is a hydrogel of a
polymer bearing randomly dispersed cationic and anionic repeat
groups.
The PA hydrogel is selected from the group consisting of random
polymers obtained by random polymerization of a cationic monomer
and an anionic monomer. The cationic monomer is selected from the
group consisting of monomers having an amino group, preferably a
quarternized amine group. Examples of the cationic monomer include
the monomer selected from the group consisting of
3-(methacryloylamino)propyltrimethylammonium chloride (MPTC),
acryloyloxethyltrimethylammonium chloride (DMAEA-Q),
methacrylatoethyl trimethyl ammonium chloride (MTAC) and
4-vinylpyridine chloride (4-VPC).
The anionic monomer is selected from the group consisting of a
sulfonate group, a sulfonic acid group, a carboxylate group, a
carboxyl acid group, a phosphate group, and a phosphoric acid
group. Examples of the anionic monomer include the monomer
selected from the group consisting of sodium p-styrenesulfonate
(NaSS), anionic 2-acrylamido-2-methylpropanesulfonic acid (AMPS),
sodium 4-styrenecarboxylic acid (NaSA), acrylic acid(AA), sodium
acrylate, methacrylic acid(MAA), and ethylene glycol
1-methacrylate 2-phosphate.
One example of the PA hydrogels is P(NaSS-co-MPTC) hydrogels,
randomly copolymerized from concentrated aqueous solutions of
oppositely charged monomers, sodium p-styrenesulfonate (NaSS) and
3-(methacryloylamino)propyltrimethylammonium chloride (MPTC). One
example of the P(NaSS-co-MPTC) hydrogels exhibits high strength
(tensile fracture stress ob . 1.8 MPa), high extensibility
(tensile fracture strain eb = 7.4), and high toughness (tearing
energies T = 4000 J m<"2>). The PA hydrogels are
self-recoverable due to the non-covalent ionic bonds. In addition
to these excellent mechanical properties, these PA hydrogels have
excellent biocompatibility and anti-biofouling properties. [NP-6].
Another example of a PA hydrogels is the PA P(NaSS-co-DMAEA-Q)
hydrogels synthesized from sodium p-styrenesulfonate (NaSS) and
acryloyloxethyltrimethylammonium chloride (DMAEA-Q). DMAEA-Q is
relatively hydrophilic in comparison with the cationic monomer
MPTC.
The chemical structures of monomers are shown below:
Cationic monomers: MPTC, DMAEA-Q;
Anionic monomers: NaSS, AMPS.
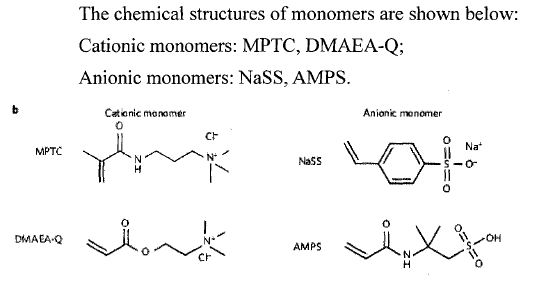
Other examples of PA hydrogels are the P(NaSS-co-MTAC4) and
P(NaSS-co-VPC) systems synthesized by using methacrylatoethyl
trimethyl ammonium chloride (MTAC) or 4-vinylpyridine chloride
(4-VPC) as a cationic monomer. Chemical structures of the cationic
monomers for the PA hydrogels are shown below:
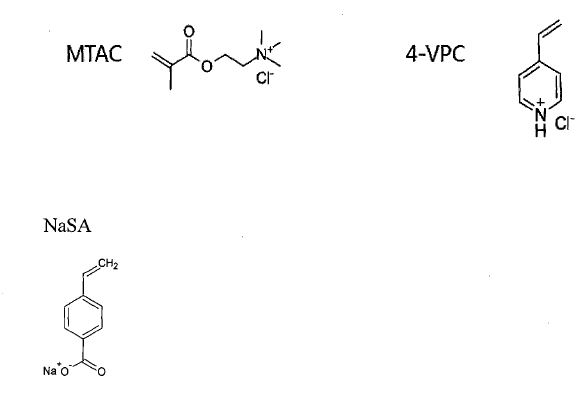
NaSA
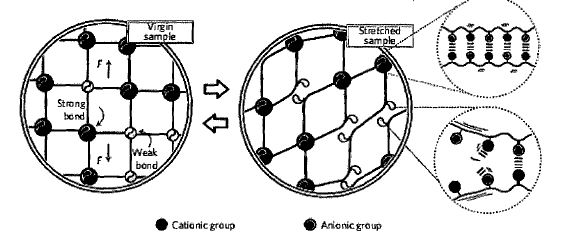
The above PAs are those developed from linear PAs by employing the
ionic bond as a reversible sacrificial bond that breaks and
reforms dynamically. [NP-6, 7] Owing to the random distribution,
the opposite charges on the PAs form multiple ionic bonds of wide
distribution in strength. The strong bonds work as permanent
cross-linkers, imparting the elasticity of the hydrogel. The weak
bonds are fragile and they break under stress, serving as
reversible sacrificial bonds. Upon rupture of the weak bonds, the
globule polymer chains unfolded, serving as hidden length. These
two processes dissipate energy and impart toughness to the
hydrogel. The PA hydrogels used in the present invention are
supramolecular hydrogels and contain about 50 wt% water, yet are
very tough and viscoelastic. An illustration of PA networks with
ionic bonds of different strength is shown below. The strong bonds
serve as permanent crosslinking points, and the weak bonds act as
reversible sacrificial bonds that rupture under deformation.
Neutral PAs form globule structures in aqueous medium by formation
of ionic bonds, which leads to phase separation. It is better to
determine the optimized charge ratio of the precursor solution to
form the neutral PA. The effects of the monomer concentration Cm
and the chemical cross-linker density C (CMBAA in case MBAA is
used as the chemical cross-linker) on the behavior of the PA is
considered when the PA hydrogel is prepared. These two parameters
are crucial to control the phase separation of the system. It is
preferred that a phase diagram of formulation in the Cm-CMBAA
space can be constructed in advance and homogenous hydrogel phase
is determined. The mechanical properties of PA hydrogels are
dependent on the combinations of the ionic monomer pairs. There is
a tendency of that more hydrophilic monomers produced softer and
stretchable gels and vice versa.
The polymers are obtained by random copolymerization of a cationic
monomer and an anionic monomer and a neutral monomer. The addition
of the neutral monomer can adjust the stiffness and toughness of
the samples. Examples of the neutral monomer include acrylamide
based monomers such as acrylamide (AAm), N-isopropylacrylamide
(NIPAM), dimethylacrylamide (DMAAm); vinyl based monomers such as
vinyl acetate, vinyl pyridine, styrene; alkylacrylate based
monomers such as methylmethacrylate; hydroxyalkyl acrylate based
monomers such as hydroxyethylacrylate; and fluorine containing
unsaturated monomers such as trifluoroethyl acrylate.
Synthesis of PA hydrogels and preparation of the Composite
PA hydrogels are synthesized using a one-step random
copolymerization of an anionic monomer and a cationic monomer.
Using a one-step random copolymerization, the composite of the
present invention can be manufactured in one step.
The present invention further relates to a method of preparation
of a composite comprising a fabric and a polyampholyte hydrogel.
The method comprises the following steps (a) to (c).
(a) providing a monomer mixture for preparation of a polyampholyte
hydrogel;
(b) immersing a fabric in the monomer mixture solution; and
(c) polymerizing monomers in the monomer mixture solution to
obtain a precursor of the composite.
(a) Provision of a monomer mixture
The monomer mixture can be a mixed aqueous solution of an anionic
monomer and a cationic monomer and the aqueous solution is
prepared by mixing the monomers with water. The monomer
concentration, Cm, influences the behavior of the resulting PA and
the preparation of the PA hydrogels. The monomer concentration,
Cm, means the total concentration of the anionic monomer and a
cationic monomer. The monomer concentration, Cm, is desirably in a
range from 1 to 5 mol/L, preferably range from 1.5 to 2.5 mol/L in
order to prepare PA hydrogels enabling to provide a tough and
stiff, yet<'>flexible composite.
In addition, molar fraction of the anionic monomer F influences
the behavior of the resulting PA and the preparation of the PA
hydrogels. The molar fraction of the anionic monomer F means a
molar fraction of the anionic monomer to the total of the anionic
monomer and a cationic monomer. The molar fraction of the anionic
monomer F is desirably in a range from 0.4 to 0.6, preferably in a
range from 0.5 to 0.54 in order to prepare PA hydrogels that
provide tough and stiff, yet flexible composites.
The mixed aqueous solution can further contain a polymerization
initiator and/or a cross-linking agent and/or a salt. The
polymerization initiator can be ultraviolet initiator such as
2-oxoglutaric acid, sodium
4-[2-(4-morpholino)benzoyl-2-dimethylamino]butylbenzenesulfonate,
and phenacylpyridinium oxalate. The concentration of the
polymerization initiator can be set in view of the kind of the
monomers and the preferable initiator concentration is, but not
limited to, in a range from 0.01 mol% to lmol% against to total
monomer concentration.
The cross-linking agent is preferably a chemical cross-linker
having two or more of (meth)acrylate groups in a molecule such as
N, N'-methylenebis (acrylamide) (MBAA) and
ethyleneglycoldimethacrylate. N, N'-methylenebis (acrylamide)
(MBAA) is preferred when an (meth)acrylate based monomer is used.
Ethyleneglycoldimethacrylate is preferred when a vinyl monomer
such as NaSS and 4-VPC is used. The cross-linking agent can be
used in combination or alone. The concentration of the
cross-linking agent can vary in view of the monomers and the
preferable concentration is, but not limited to, in a range of
0.1mol% to 10mol% against to total monomer concentration.
The salt can be an inorganic salt. Examples of the inorganic salt
include an alkali metal salt such as LiCl, NaCl, KC1, CsCl, CaCl2,
MgS04, K2S04, or MgCl2. The concentration of the salt can vary in
view of the kind of the monomers and the monomer concentration Cm
and is not limited specifically but is in a range of 0.1M to 2M,
for example. The usage of salt can reduce the viscosity of the
mixed aqueous solution and obtain the homogeneous samples.
However, the higher the salt concentration, the higher the burden
of removal of the salt after polymerization.
(b) Immersion of a fabric into the monomer mixture solution; A
cell for preparation of the composite is comprised of, but not
limited to, a pair of plates such as glass plates facing each
other and the thickness between the plates is controlled by a
spacer thickness. This spacing is, but not limited to, desirably
in a range from 0.001 mm to 10 mm, preferably in a range from 0.01
mm to 5.0 mm, and more preferably in a range from 0.1 mm to 1.0
mm. The plates and the spacer are selected from the material inert
with the mixed aqueous solution and the spacer can be a silicone
spacer, for example, with a prescribed thickness. The thickness of
the spacer decides the thickness of the composite. A fabric is
placed in the space made by the plates. This fabric can be woven
or knit or unwoven and may range in thickness from 0.001 mm to 1
mm in thickness, and may consist of a plain, twill, or satin
weave. The mixed aqueous solution is added into the channel in
which the fabric has been placed. The fabric can be placed
nominally in the center of the space, or preferentially towards an
edge or surface such as 60/40, 70/30, 80/20, etc. distribution of
the PA on each side of the fabric. Alternatively, the composite
may be prepared by coating the fabric with the mixed aqueous
solution on either or both sides of the fabric surface. The
coating may be applied by spraying, dipping, knife-over-roll, or
other traditional fabric coating methods.
(c) Polymerization of the monomers to obtain a precursor of the
composite The mixed aqueous solution is cured, for example, by
irradiation of ultraviolet light when an ultraviolet initiator is
used. Wavelength of the ultraviolet light can be determined in
view of the ultraviolet initiator. The irradiation can be
conducted in an inert atmosphere, such as argon atmosphere, at an
ambient temperature or an increased temperature for 1 minute to 24
hours, preferably from 1 hour to 12 hours, and more preferably
from 8 hours to 12 hours, which is adjusted by the power of
ultraviolet light. This reaction can take place at an ambient
temperature or an increased temperature in an inert atmosphere
such as an argon or nitrogen atmosphere. The composite consisted
of fabric and PA can be prepared with the thickness from 0.001mm
to 10mm controlled by the size of spacer.
In this method, the cationic and anionic monomers are essentially
copolymerized randomly in the PA hydrogels. The random structure
of the copolymer can be confirmed using a Ή-NMR reaction kinetics
study, for example.
After polymerization, the as-prepared gel is immersed in water to
reach equilibrium and to wash away the residual chemicals after
one week, for example. The amount of the water is not limited
specifically and it is preferred to confirm the equilibrium state
and disappearance of the residual chemicals in the gel. During
this process, the mobile counter-ions of the ionic copolymer as
well as the salt used, are removed from the gel, and the
oppositely charged ions on the copolymer form stable ionic
complexes either through intra- or interchain interactions.
Polymerization conditions and structural features and mechanical
properties of various PA hydrogels are shown in the table below.
Table 1
* Cm and / represent the total ionic monomers concentration
(mol/L) and the charge fraction of the anionic monomer,
respectively, in the precursor solution used to synthesize the
gels. The parameters cw, E, ab, eb, Wb, tan5, and R are the water
content, Young's modulus, fracture stress, fracture strain, work
of extension at fracture, loss factor (10 Hz and strain 0.5%), and
shock-absorbing ratio, respectively, at room temperature. 7s is
the softening temperature determined by the peak of loss factor of
the gels.
A computer-aided drawing of one embodiment of the composites of
the present invention is shown in Figure 1. Left side is a side
view of the composite and right side is a projection view. One
embodiment of the composites of the present invention exhibits
toughness two orders of magnitude higher than traditional
hydrogels (SN gel) (Gc 4800 J/m<2>vs 10 J/m<2>). In
addition, one embodiment of the composites of present invention
exhibits dramatic increase in toughness. For the case of a 20 mm
wide strip of the composite comprised of woven glass fiber and PA
gel, a Gc value (105,000 J/m<2>) can be obtained which is an
additional two orders of magnitude greater than the neat PA gel,
and 4 orders of magnitude higher than a traditional SN gel. (See
Figure 3).
Interestingly, unlike most composites where combining two
materials results in an averaging of their mechanical properties,
in this case the tear strength is much greater than either
materials when tested independently. Also when the control SN gel
and fabric was tested, a noticeable decrease in tear strength was
observed, which reinforces that this special combination of PA gel
and fabric is necessary to see a great increase in tear strength
(See the following Examples).
The composite of the present invention can be employed for medical
treatment for example for ligament reconstruction surgeries such
as Anterior Cruciate Ligament (ACL) reconstruction surgeries. The
composite of the present invention can also be employed for
bandages, skin grafts, flexible tear resistant clothing or
bullet-proofing.
There are many ways this invention improves over the prior art.
When used as internal prostheses, fabrics have many issues with
regards to tearing, failing, and degrading. Often to prevent this,
these stiff substrates would be coated in elastomers. However
elastomers often result in a bodily response which can lead to
infection or rejection. The coating material, the PA hydrogel,
used here contains primarily of water and is non-toxic, and
resists cell adhesion.
The composite of the present invention has various utilities.
Wound care would benefit from this invention, because the PA
hydrogels could create extremely resilient and tear resistant
bandages. In the composite of the present invention, the fabric is
used as support and the PA hydrogel is used for wound closure, as
they are generally non-toxic, consist mostly of water, and can
promote wound healing.
Examples
The present invention will be described in detail below based on
examples. However, the present invention is not limited to the
examples.
1. Materials
Sodium p-styrenesulfonate (NaSS), Methyl chloride quarternized N,
N-dimethylamino ethylacrylate (DMAEA-Q), Acrylamide (AAm),
2-oxoglutaric acid and N, N'-methylenebis (acrylamide) (MBAA) were
purchased from the Wako company (Japan). Fabric (S-Glass 4-Harness
Satin Weave) (4HS) were purchased from the ACP composite company.
In the 4HS, the fill yarn passes over three warp yarns and under
one. All of the chemicals were used as purchased without further
purification.
2. Methods
2- 1. Synthesis of materials
Polyampholyte hydrogels (PA) were synthesized using the one-step
random copolymerization of an anionic monomer NaSS and a cationic
monomer DMAEA-Q. A mixed aqueous solution with the prescribed
monomer concentration (Cm=2.0 mol/L) and molar fraction of the
anionic monomer (F=0.52), 0.1mol% ultraviolet initiator,
2-oxoglutaric acid, 0.1mol% chemical crosslinker MBAA (in a
concentration relative to the total monomer concentration), and
0.5M NaCl was poured into a reaction cell. The cell was consisted
of a pair of glass plates with 10 mm (L)x l0 mm (w)x lmm(d), where
the thickness was controlled by the silicone spacer thickness. The
reacted system was irradiated with 365-nm ultraviolet light for 8h
in the atmosphere of argon.
Polyacrylamide hydrogels (PAAm) were produced from the
polymerization of 2 mol/L acrylamide monomer, 0.1mol%
2-oxoglutaric acid and 1.0mol% chemical crosslinker MBAA (relative
to the total monomer concentration) under the UV light.
PA gel/fabric composite were produced by the similar described
polymerization process. The fabric 4HS with approximately 0.3 mm
thickness were fixed to the center of reaction cell prior to
injecting the reaction solution. To produce the PA gel/fabric
composite, the polymerization was carried out after filling the PA
solution with the same composition to the reaction cell.
Similar to the PA gel/fabric composite production, the PAAm/fabric
composite was synthesized by the AAm solution and fabric 4HS.
[0059]
After polymerization, the as-prepared gel was immersed in a large
amount of water for 1 week to allow the gel to reach equilibrium
and to wash away the residual chemicals and counter-ions.
2-2. Characterization
Tearing test:
The tearing test was performed to characterize the toughness in
air using a commercial test machine (Instron Cat. No 2752-005).
The image of testing setup for the tearing test is shown in Figure
2. The test samples were cut to rectangular shape with 40 mm (L) x
20-100 mm (w)<χ>0.5-1.3 mm (t). A cut was made 20 mm along
the length, at the center of the width of the sample, to create
two arms. The two arms of a test piece were clamped, and then the
upper arm was pulled upward at constant velocity 50 mm/min while
the tearing force F was recorded. The tearing energy T was
calculated at a constant tearing force F using the relation T =
2F/w, where w is the thickness of the sample. The results are
shown in Figure 3 and 4. Figure 3 shows tear strength at breakage
and Figure 4 shows force vs. displacement curve for 20 mm wide
samples of the composite of the present invention, a neat glass
fiber fabric, a combination of the fabric and a SN hydrogel.
Figure 5 shows microscopy images of glass fiber fabric and PA
hydrogel between the fibers of the fabric of the sample, A is an
image of fibrillation in the matrix gel and B is an image of
fracture of the fibrils, which is hypothesized to dissipate
energy. Figure 6 is a microscopy image showing deformation in the
tearing region of the fabric (macro scale).
Tensile test:
The tensile stress-strain measurements were performed using a
tensile-compressive tester (Tensilon RTC-1310A, Orientec Co.) at a
deformation rate of 10 mm/min in air. The test was carried out on
samples with rectangle shape with the size (25 mm (L) x 20 mm
(w)<χ>0.85 mm (d). In order to prevent the slipping of the
sample at the end of the clamp during the tensile test,
polyethylene terephthalate (PET) plates with 2 mm (L) x 2 mm
(w)<χ>3mm (d) adhere the ends of samples was used by super
glue cyanoacrylate. The PET plates were gripped by the clamp
during the tensile test. The results are shown in Figures 7
(composite) and 8 (neat glass fabric). The strength of the
materials exceeded the strength of the mounting grips, and the
ultimate properties of these materials exceed the results
presented. [0062]