
rexresearch.com
John GOODENOUGH, et al.
Glass Battery
https://news.utexas.edu/2017/02/28/goodenough-introduces-new-battery-technology
Lithium-Ion Battery Inventor Introduces New
Technology for Fast-Charging, Noncombustible Batteries
A team of engineers led by 94-year-old John Goodenough, professor
in the Cockrell School of Engineering at The University of Texas
at Austin and co-inventor of the lithium-ion battery, has
developed the first all-solid-state battery cells that could lead
to safer, faster-charging, longer-lasting rechargeable batteries
for handheld mobile devices, electric cars and stationary energy
storage.
Goodenough’s latest breakthrough, completed with Cockrell School
senior research fellow Maria Helena Braga, is a low-cost
all-solid-state battery that is noncombustible and has a long
cycle life (battery life) with a high volumetric energy density
and fast rates of charge and discharge. The engineers describe
their new technology in a recent paper published in the journal
Energy & Environmental Science.
“Cost, safety, energy density, rates of charge and discharge and
cycle life are critical for battery-driven cars to be more widely
adopted. We believe our discovery solves many of the problems that
are inherent in today’s batteries,” Goodenough said.
The researchers demonstrated that their new battery cells have at
least three times as much energy density as today’s lithium-ion
batteries. A battery cell’s energy density gives an electric
vehicle its driving range, so a higher energy density means that a
car can drive more miles between charges. The UT Austin battery
formulation also allows for a greater number of charging and
discharging cycles, which equates to longer-lasting batteries, as
well as a faster rate of recharge (minutes rather than hours).
Today’s lithium-ion batteries use liquid electrolytes to transport
the lithium ions between the anode (the negative side of the
battery) and the cathode (the positive side of the battery). If a
battery cell is charged too quickly, it can cause dendrites or
“metal whiskers” to form and cross through the liquid
electrolytes, causing a short circuit that can lead to explosions
and fires. Instead of liquid electrolytes, the researchers rely on
glass electrolytes that enable the use of an alkali-metal anode
without the formation of dendrites.
The use of an alkali-metal anode (lithium, sodium or potassium) —
which isn’t possible with conventional batteries — increases the
energy density of a cathode and delivers a long cycle life. In
experiments, the researchers’ cells have demonstrated more than
1,200 cycles with low cell resistance.
Additionally, because the solid-glass electrolytes can operate, or
have high conductivity, at -20 degrees Celsius, this type of
battery in a car could perform well in subzero degree weather.
This is the first all-solid-state battery cell that can operate
under 60 degree Celsius.
Braga began developing solid-glass electrolytes with colleagues
while she was at the University of Porto in Portugal. About two
years ago, she began collaborating with Goodenough and researcher
Andrew J. Murchison at UT Austin. Braga said that Goodenough
brought an understanding of the composition and properties of the
solid-glass electrolytes that resulted in a new version of the
electrolytes that is now patented through the UT Austin Office of
Technology Commercialization.
The engineers’ glass electrolytes allow them to plate and strip
alkali metals on both the cathode and the anode side without
dendrites, which simplifies battery cell fabrication.
Another advantage is that the battery cells can be made from
earth-friendly materials.
“The glass electrolytes allow for the substitution of low-cost
sodium for lithium. Sodium is extracted from seawater that is
widely available,” Braga said.
Goodenough and Braga are continuing to advance their
battery-related research and are working on several patents. In
the short term, they hope to work with battery makers to develop
and test their new materials in electric vehicles and energy
storage devices.
John Goodenough
Maria Braga
http://pubs.rsc.org/en/content/articlelanding/2016/ee/c5ee02924d#!divAbstract
Energy Environ. Sci., 2017,10, 331-336
DOI: 10.1039/C6EE02888H
Alternative strategy for a safe
rechargeable battery
M. H. Braga, N. S. Grundish, A. J. Murchisona, J. B.
Goodenough
The advent of a Li+ or Na+ glass electrolyte with a cation
conductivity σi > 10−2 S cm−1 at 25 °C and a motional enthalpy
ΔHm = 0.06 eV that is wet by a metallic lithium or sodium anode is
used to develop a new strategy for an all-solid-state,
rechargeable, metal-plating battery. During discharge, a cell
plates the metal of an anode of high-energy Fermi level such as
lithium or sodium onto a cathode current collector with a
low-energy Fermi level; the voltage of the cell may be determined
by a cathode redox center having an energy between the Fermi
levels of the anode and that of the cathode current collector.
This strategy is demonstrated with a solid electrolyte that not
only is wet by the metallic anode, but also has a dielectric
constant capable of creating a large electric-double-layer
capacitance at the two electrode/electrolyte interfaces. The
result is a safe, low-cost, lithium or sodium rechargeable battery
of high energy density and long cycle life.
US2016368777
WATER SOLVATED GLASS/AMORPHOUS SOLID IONIC CONDUCTORS
Inventor: GOODENOUGH JOHN, et al.
TECHNICAL FIELD
[0002] The disclosure provides a dried, water-solvated
glass/amorphous solid that is an alkali-ion conductor and an
electronic insulator with a large dielectric constant. The
disclosure also provides electrochemical devices and processes
that use this material, such as batteries, including rechargeable
batteries, fuel cells, capacitors, electrolytic generation of
chemical products, including hydrogen gas (H2), from water, and
electronic devices. The electrochemical devices and products use a
combination of ionic and electronic conduction. The disclosure
also provides a water-solvated glass/amorphous solid that is a
proton (H<+>) conductor and an electronic insulator.
BACKGROUND
[0003] Ionic conductors that are also electronic insulators are
called electrolytes; they may be a liquid or a solid. Electrolytes
are used in a variety of electrochemical devices, including not
only those that store electric power as chemical energy in a
rechargeable battery or those that release chemical energy as
electric power in a fuel cell, but also those that store electric
power as static electric energy in an electric-double-layer
capacitor. Electric power that is released from an electric-energy
store, whether from a chemical or an electrostatic store, is clean
energy. Chemical energy stored in a fuel that is released as the
heat of combustion is a less efficient process, and combustion is
also accompanied by the release of gases that pollute the air and
contribute to global warming.
[0004] An electrochemical cell contains an electrolyte between two
electrodes, an anode and a cathode. A liquid electrolyte requires
use of a separator of the two electrodes that is permeable by the
liquid electrolyte; the separator prevents electronic contact
between the two electrodes within the cell. A solid electrolyte
may serve as both an electrolyte and a separator. In a
rechargeable battery, the anode is a reductant; in a fuel cell,
the anode catalyzes the separation of a reductant fuel into its
electronic and ionic components. In both types of cells, the ionic
component of the chemical reaction between two electrodes is
transported to the cathode inside the cell in the electrolyte, but
the electrolyte forces the electronic component to go to the
cathode via an external circuit as an electronic current I at a
voltage V to provide electric power P=IV for performance of work.
Since the ionic conductivity in the electrolyte is much smaller
than the electronic conductivity in a good metal, battery cells
and fuel cells are fabricated with large-area electrodes and a
thin electrolyte; the active electrode materials are fabricated to
make electronic contact with a metallic current collector for fast
transport of electrons between the active electrode particles and
the external circuit as well as ionic contact with the electrolyte
that transports ions between the electrodes inside the cell.
[0005] Solid electrolytes with a large dielectric constant may
also be used in electronic devices as separators of liquid or
gaseous reactants as well as of solid reactants.
[0006] Liquids are generally much better ionic conductors at room
temperature than most known solids, which is why liquids are
normally used as the electrolyte of a room-temperature device.
However, in some applications a solid electrolyte may be strongly
preferred. For example, the Li-ion rechargeable battery uses a
flammable organic liquid as the electrolyte, and a solid
electrolyte would be safer and might be capable of improving the
density of energy stored without sacrificing the rate of charge
and discharge. Moreover, if the solid electrolyte also contains
electric dipoles that give it a high dielectric constant, it can
store much more electric energy than a liquid in an electric
capacitance of an electric double layer of a metal/electrolyte
interface.
[0007] In an electric-double-layer capacitor, metallic electrodes
are fabricated so as to provide a maximum electrode/electrolyte
interface. Ions in the electrolyte pin electrons or electron holes
of opposite charge in the electrode across an electric double
layer on charge. The separation of the electrons and holes across
the double layer is small (atomic dimension) so the capacitance is
large. On discharge, pinned electrons at the anode pass through
the external circuit to recombine with the pinned electron holes
in the cathode, and the mobile ions inside the electrolyte return
to an equilibrium position. If the electrolyte has a large
dielectric constant εE′r, the capacitance of the electric double
layer is enhanced. With a solid electrolyte having a large
dielectric constant, the enhancement of the capacitance is large,
and it becomes possible to construct a cell where the energy
stored has a Faradaic component as in a battery and a capacitive
component as in an electric-double-layer capacitor.
SUMMARY
[0008] The present disclosure inlcudes a dried, water-solvated
glass/amorphous solid electrolyte that conducts either Li<+>
or Na<+>, or both, nearly as rapidly as a flammable organic
liquid at room temperature and also has a large dielectric
constant. Moreover, alkali metals can be plated and stripped
from/to it without dendrite formation, thus avoiding safety issues
and a limited charge/discharge cycle life. A dried, water-solvated
glass/amorphous solid that conducts Li<+ >may be referred to
herein as a “Li-glass.” A dried, water-solvated glass/amorphous
solid that conducts Na<+>may be referred to herein as a
“Na-glass.”
[0009] The present disclosure inlcudes a water-solvated
glass/amorphous solid electrolyte that conducts H<+> and may
be referred to herein as a “proton electrolyte.”
BRIEF DESCRIPTION OF THE DRAWINGS
[0010] A more complete understanding of the present embodiments
and advantages thereof may be acquired by referring to the
following description taken in conjunction with the accompanying
drawings, which relate to embodiments of the present disclosure.
[0011] FIG. 1 is a graph comparing Arrhenius plots of
Lithium-ion (Li<+>) conductivity (σLi) versus temperature
of a polymer gel with a salt, LiPF6, and that of a Li-glass
formed from precursor lithium hydroxides, LiOH, chlorides, LiCl
and solvated water (H2O); the solid was dried before
measurement. The conductivity of AgI is also shown.
[0012] FIG. 2 is a graph showing the dependence on
temperature, closed circles, and time at 25° C., open circles,
of the Na<+ >conductivity, σNa, of a Na-glass.
[0013] FIG. 3 is a graph showing the temperature dependence
of the relative permittivity (ε=ε′+iε″) measured in an ac field
of frequency f=1000 Hz, of a Li-glass obtained from a precursor
composition of nominal Li2.9Ba0.005ClO. ε′ is the dielectric
constant.
[0014] FIG. 4A is an Arrhenius plot showing the temperature
dependence of the proton (H<+>) conductivity (σH) of a
proton electrolyte solid obtained by solvating water in BaKPO4.
[0015] FIG. 4B is a graph showing a representative Nyquist
plot taken at 25° C. of the frequency dependence of σH of a
proton electrolyte; the impedance is Z=Z′+iZ″.
[0016] FIG. 5 is a graph showing the charge/discharge
cycling of a capacitor formed by a thick, Li-glass electrolyte
sandwiched between two aluminum plates.
[0017] FIG. 6 is a schematic diagram of the ordering with
time, pressure, and/or temperature of electric dipoles in an ac
or dc electric field.
[0018] FIG. 7 is a graph showing charge/discharge curves of
a full lithium cell showing plating/stripping of a metallic
lithium anode from a Li-glass electrolyte.
[0019] FIG. 8 is a graph showing charge/discharge voltages
of a full sodium cell showing plating/stripping of a
metallic-sodium anode from a Na-glass electrolyte.
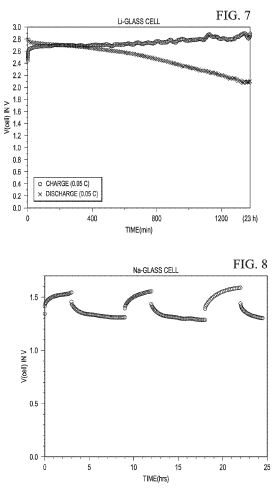
DETAILED DESCRIPTION
[0020] The present disclosure relates to a water-solvated
glass/amorphous solid that conducts monovalent cations such as
Li<+>, Na<+>, or H<+>, and mixtures thereof, and
is an electronic insulator. If the water-solvated glass/amorphous
solid conducts Li<+>, Na<+>, or mixtures thereof, it
is dried; an H<+> conductor is not dried. The Li-glass and
Na-glass are excellent conductors of Li<+>, Na<+ >or
mixtures thereof, and have high dielectric constants because of
the presence of electric dipoles. They also have a large enough
electronic-state energy gap not only to be excellent electronic
insulators, but also to allow plating of alkali-metal anodes and
the use of high-voltage cathodes in alkali-metal rechargeable
batteries that contain the dried water-solvated glass/amorphous
solid as the electrolyte or separator; electrochemical capacitors
of high electrical-storage capacity can also be made with the
Li-glass or Na-glass as the electrolyte. They are wet by the
alkali metal to allow plating and stripping of alkali-metal anodes
without dendrite formation, and they are capable of high-voltage
storage of electrostatic energy at a glass/metal interface. The
materials can be formed as a paste for facile application to a
large surface area. They can be used as the electrolyte and/or
separator of a battery, fuel cell, or electrolysis cell and/or as
a material in a capacitor of an electronic device.
[0021] The disclosure also includes a method of forming the
water-solvated glass/amorphous solid electrolyte from constituent
precursors containing at least one alkali metal atom, particularly
lithium (Li) and/or sodium (Na), with oxygen and/or at least one
halide atom, particularly chlorine (Cl), bromine (Br), iodine (I),
or mixtures thereof, and water (H2O) added in an amount less than
or equal to the solvation limit of the glass/amorphous product.
For example, the constituent precursors of the glass/amorphous
product may include A3-xHxOX, AX+A2O, or 2AOH+AX (H2O) with x≦1
where A is an alkali metal such as Li and/or sodium Na or a
mixture thereof and X a halide atom. The constituent precursor may
also contain an oxide or hydroxide promotor of glass formation
such as Ba(OH)2, Sr(OH)2, BaO, SrO, CaO, MgO, Al2O3, B2O3, or SiO2
and a promoter in which sulfur replaces the oxygen. An alternative
is to press at an appropriate temperature the precursor oxide,
hydroxide, halide, and any other additive, including H2O, until it
forms a glass.
[0022] In addition, the disclosure includes a method of drying the
water-solvated glass/amorphous product. The method makes use of
two chemical reactions. First, the reaction
H2O+X<−>=(OH)<−>+HX↑, where HX evaporates as a gas,
e.g. HCl, during heating to form the glass/amorphous product.
Second, the reaction 2(OH)<−>=O<2−>+H2O↑ exhausts
steam (gaseous H2O) below the decomposition temperature of the
glass.
[0023] Excess alkali ions (A<+>) can form three types of
dipole to give a large dielectric constant: OH<−>,
OA<−>, and A<+ >in an asymmetric glass anion site.
Orientation of the dipoles at higher temperatures, e.g.
50<T<110° C., in an ac or dc electric field before cooling
to room temperature may be used to optimize more rapidly the
cation conductivity at room temperature.
[0024] The disclosure also includes a method of fabricating the
dried glass/amorphous product as a thin electrolyte in a cell
where it separates two electrodes. The method includes breaking
the glass/amorphous product into small pieces and an aprotic
liquid, such as ethylene carbonate (EC), added to aid compaction
of the powder into a dense film covering a current collector or an
alkali metal anode that, on heating, reforms into a thin, dry
glass/amorphous film with no grain boundaries.
[0025] Alternatively, the dry glass/amorphous product may be
ground to small particles in an aprotic liquid such as ethanol to
form a slurry or ink that can be applied as a thin layer over a
large area of arbitrary shape; by a convenient method such as
doctor-blading, printing, or vapor deposition. The cell ensemble
is then sealed by a sealant such as Epoxy that cures exothermally
and remains permeable to the evaporating gas from the liquid of
the slurry while it is wet, but becomes impermeable as a solid
sealant once it dries. Alternatively, the glass may be dried in a
dry room. During evaporation of the liquid of the slurry, the
glass/amorphous particles reform without grain boundaries into a
continuous sheet as a Li-glass or Na-glass electrolyte having a
large dielectric constant owing to the presence of electric
dipoles.
[0026] The disclosure also includes a water-solvated
glass/amorphous proton (H<+>) electrolyte formed by
solvating water into a crystalline solid containing a strongly
electropositive cation such as a large alkali ion like that of
potassium (K<+>), rubidium (Rb<+>), or cesium
(Cs<+>) and a strongly acidic polyanion such as
(SiO4)<4−>, (PO4)<3−>, or (SO4)<2−>. The
solvated water, H2O, is captured by the strongly electropositive
cations as an (OH)<− >ligand with the release of the
H<+> ion, which is mobile in the presence of the solid
polyanions. This process transforms the crystalline parent
compound into a proton electrolyte.
[0027] The disclosure includes a water-solvated glass/amorphous
solid produced by any of these methods.
[0028] The disclosure also relates to a paste including particles
of a Li-glass or N-glass as described above in an organic liquid,
an ionic liquid, and/or a polymer.
[0029] The disclosure further includes dielectric
electrolytes-formed from a water-solvated glass/amorphous solid or
paste as described above.
[0030] The disclosure additionally includes a method of forming a
dielectric electrolyte by forming a paste as described above,
applying the paste to a surface, and allowing some or all of the
organic liquid, ionic liquid, and/or polymer to evaporate, leaving
a reformed electrolyte dielectric. The disclosure includes the
electrolyte-dielectric thus formed.
[0031] A water-solvated, dried glass/amorphous alkali-ion
electrolyte having a large dielectric constant that may be used in
an electrochemical cell that stores electric power as in a
rechargeable battery, a cell that stores electric power as static
electricity in the capacitances of an electric double layer at a
metal/electrolyte interface, a cell that accomplishes both types
of electric-power storage in the same cell, or a cell that is used
in an electronic device.
Electrolyte/Dielectric Material
[0032] The water-solvated dried glass/amorphous solid may be
formed from a crystalline electronic insulator or its constituent
precursors (e.g. LiCl+2Li(OH)+xBa(OH)2.8H2O) by the addition of
water (H2O) up to the solubility limit of the crystalline
electronic insulator. Water is solvated into the crystalline
electronic insulator by separation of the hydroxide (OH<−>)
anion from the proton. Where this separation occurs, the solvated
water acts like a salt dissolved in a liquid. The separation of
the hydroxide anion and the proton may be stabilized by trapping
the proton by an X<− >ion with the escape of HX gas; and
mobile OH<− >ions may react with one another to form H2O
that leaves the solid at higher temperature. The separation of the
H<+> and (OH)<− >ions may also be achieved by the
trapping of OH<−> anions at a large, strongly
electropositive atom like Ba<2+>, K<+>, Rb<+>,
Ca<+ >with the release of the H<+> ion to an acidic
matrix.
[0033] If a halide (X<−>) anion, such as a chloride
(Cl<−>) anion, a bromide (Br<−>) anion, and/or an
iodide (I<−>) anion, is also present in the crystalline
electronic insulator, the proton can combine with the X<−
>anion and depart from the solid as a hydrogen halide (HX) gas,
with the hydroxide anion remaining in the solid. The mobile
OH<− >ions may react with one another to form O<2−
>and H2O with the water leaving the solid at higher
temperatures. The departure of the proton (H<+>) and water
from the water-solvated glass/amorphous solid means that the
product is dry and can be used to contact an alkali-metal anode in
a battery or in other electronic devices sensitive to the presence
of water. If the hydroxide anions are not trapped in a hydrated
polyanion such as Ba(OH)x<(2-x)>, they are mobile, as are
any alkali cations, such as lithium ion (Li<+>) and/or
sodium ion (Na<+>), of the-electronic insulator. The lithium
ion (Li<+>) and/or sodium ion (Na<+>) are much more
mobile than the OH<− >anions. Nevertheless, the mobile
(OH)<− >ions may react as 2(OH)<−>=O<2−>+H2O↑
with the escape of steam at higher temperatures.
[0034] Alternatively, if a large cation like the barium ion
(Ba<2+>) or potassium ion (K<+>) rubidium
(Rb<+>), or cesium (Cs<+>) is present in a crystalline
electronic insulator, the hydroxide (OH<−>) anion of the
solvated water (H2O) may be trapped in a-polyanion of the large
cation and the proton (H<+>) may be mobile if the other
anion of the crystalline electronic insulator is a strongly acidic
polyanion like phosphate (PO4)<3− >or sulfate
(SO4)<2−>. Most of the protons (H<+>) are not trapped
by the polyanions or in a hydrogen bond so long as the solvated
water has transformed the crystalline electronic insulator into a
water-solvated glass/amorphous solid.
[0035] The finished water-solvated glass/amorphous solid may be
derived from any crystalline electronic insulator or its mix of
oxide, hydroxide, and/or halide constituent precursors that can be
transformed into a glass/amorphous solid by the solvation of water
into it with or without the aid of an oxide, sulfide, or hydroxide
additive. If the original crystalline material contains a large
concentration of alkali ions bonded to oxide and/or halide ions,
it may be transformed into a fast conductor of lithium ion
(Li<+>) and/or sodium ion (Na<+>) and an electronic
insulator by drying at high temperatures. If the crystalline
electronic insulator contains only acidic polyanions and large,
electropositive cations that stabilize hydroxide polyanions,
transformation to a water-solvated glass/amorphous solid by the
solvation of water provides a fast proton (H<+>) conductor.
[0036] The water used to form a Li-glass or Na-glass may include
less than two mole percent water and less than one mole percent of
a glass-forming additive. The glass-forming additive may aid the
transformation of the crystalline electronic insulator into a
dried water-solvated glass/amorphous solid. The glass-forming
additive may include at least one oxide, sulfide, and/or
hydroxide, such as barium oxide (BaO), magnesium oxide (MgO),
calcium oxide (CaO) and/or barium hydroxide Ba(OH)2, Mg(OH)2,
Ca(OH)2, Sr(OH)2, or Al(OH)3, BaO, SrO, CaO, MgO, Al, B2O3, Al2O3,
SiO2, S or Li2S, and mixtures thereof. The water-solvated
glass/amorphous solid has a glass transition temperature, Tg, that
can be adjusted by the character of the cation that is introduced
into the crystalline electronic insulator or its constituent
precursor to promote glass formation. In addition, the hydroxide
(OH<−>)<−> anions of the dried water-solvated
glass/amorphous solid or any other electric dipole like (OH)<−
>or (OA)<− >where A=Li or Na, or an A<+ >ion in an
asymmetric glass site may be oriented in an ac or dc electric
field to enhance the dielectric constants and the cation
conductivity.
[0037] The water-solvated glass/amorphous solid may be ground into
a plurality of small pieces and mixed with a polymer, an ionic
liquid, and/or an organic liquids such as ethanol that evaporates
quickly or ethylene carbonate (EC) in order to form a paste for
easy application over a large surface area before reforming into a
glassy amorphous solid. This process may improve contact with a
solid electrode and/or current collector. Upon evaporation of some
or all of the liquid component, the glass/amorphous solid is
reformed as a large-volume ionic conductor with few, if any, grain
boundaries. Evaporation may occur prior to inclusion in an
electrochemical device or afterwards.
[0038] Two specific processes illustrate the transformation of the
constituent precursor of a crystalline electronic insulator into a
water-solvated glass/amorphous solid that is an ionic conductor
and electronic insulator that is dry.
[0039] (1) The constituent precursor oxides, hydroxides, and
halides of the crystalline electronic insulator may have the
general formula A3-x<+>Hx<+>OX, wherein 0≦x≦1 and A is
lithium (Li) and/or sodium (Na) and wherein X is chlorine (Cl),
bromine (Br), and/or iodine (I). This starting material is rich in
alkali ions bonded to only oxide and halide anions. Addition of
water up to the solubility limit of the water with or without the
addition of an oxide and/or hydroxide such as barium oxide (BaO),
magnesium oxide (MgO), and/or barium hydroxide (Ba(OH)2)
transforms the crystalline electronic insulator or constituent
precursor to a dry water-solvated glass/amorphous solid that is a
lithium ion (Li<+>) and/or sodium ion (Na<+>) ionic
conductor that remains an electronic insulator. The glass
transition temperature decreases with an increase of the size of
the cation of the added oxide and/or hydroxide; with the barium
ion (Ba<2+>) and lithium ion (Li<+>), a Tg≈55° C. is
obtained.
[0040] In one example, the constituent precursors of the
crystalline material Li3-xHxOCl contained an added 0.005 Barium
oxide (BaO) per formula unit. Hydrogen chloride (HCl) gas left the
solid during a moderate-temperature anneal of the water-solvated
glass/amorphous solid. Hydroxide (OH<−>) anion conductivity
was also observed, but was much smaller than lithium ion
(Li<+>) conductivity, and above 230° C., a weight loss
signaled the occurrence of the reaction
2(OH)<−>=O<2−>+H2O↑ as a result of the evaporation of
the water (H2O). FIG. 1 illustrates lithium ion (Li<+>)
conductivity as a function of temperature in an Arrhenius plot for
this material. FIG. 3 presents the variation of the dielectric
constant of this material with temperature.
[0041] FIG. 2 illustrates sodium ion (Na<+>) conductivity as
a function of temperature in an Arrhenius plot for a
water-solvated glass/amorphous solid in which sodium (Na) replaced
(Li) in the constituent precursor for Na3-xHxOCl to which 0.005
Barium oxide (BaO) per formula unit was added. Hydrogen chloride
(HCl) gas left the solid during a moderate-temperature anneal of
the water-solvated glass/amorphous solid. Hydroxide
(OH<−>)<−> conductivity was also observed, but was
much smaller than the sodium ion (Na<+>) and above 230° C.,
a weight loss signaled the reaction
2(OH)<−>=O<2−>+H2O↑ which dried completely the
glass/amorphous products.
[0042] Water-solvated glass/amorphous solid sodium-ion
(Na<+>) and lithium-ion (Li<+>) conductors have been
used to plate reversibly metallic sodium (Na) or metallic lithium
(Li) onto itself without dendrites over 1000 times, thereby
proving that a dry water-solvated glass/amorphous solid can be
used in a rechargeable sodium-ion or lithium-ion battery and that
similar dry materials can be used in other batteries or
water-sensitive devices.
[0043] (2) KH2PO4 is a crystalline ferroelectric in which the
protons (H<+>) are trapped in hydrogen bonds. However,
BaKPO4 is a crystalline electronic insulator containing large
barium ions (Ba<2+>) and potassium ions (K<+>) ions
that can stabilize hydroxide polyanions if exposed to water vapor.
Solvation of water into this solid creates a water-solvated
glass/amorphous solid that is a fast H<+ >conductor and an
electronic insulator.
[0044] FIG. 4 presents an Arrhenius plot of the proton
(H<+>) conductivity of the water-solvated glass/amorphous
solid derived from BaKPO4 by exposure to water vapor at 80° C.
Note that the proton conductivity is σH=10<−2 >S cm<−1
>at a T≈75° C., which makes it possible to use it as a
replacement for a NAFION membrane in a room-temperature fuel cell
or a rechargeable battery with a redox-couple flow-through liquid
electrode.
Electrolytes
[0045] The magnitude of the ionic conductivity of an electrolyte
in an electrochemical cell dictates the thickness and area of the
electrolyte separating the two electrodes for a desired output
current I. The energy difference Eg between the lowest unoccupied
molecular orbital (LUMO) and the highest occupied molecular
orbital (HOMO) of the electrolyte dictates the highest voltage V
for stable operation of a cell. Therefore, the electric power on
charge and discharge, Pch=IchVch and Pdis=IdisVdis, depends
critically on the electrolyte as also does the efficiency of
storage of electrical energy, 100 Pdis/Pch%. The voltages of a
cell are
Vch=Vocηch(Ich) and Vdis=Voc−ηdis(Idis) (1)
where the voltage at open electronic circuit is Voc=(μA−μC)/e; the
μA and μC are, respectively, electrochemical potentials of the
anode and the cathode, and e is the magnitude of the electronic
charge.
[0046] The ηch and ηdis are called, respectively, the overvoltage
and the polarization. The η(q)=IRcell depend on the resistances
Rcell=Rel+Rct; Rel is the resistance to the ionic conductivity
σi=niqiμi in the electrolyte and Rct is the resistance to ionic
transport across any electrode/electrolyte interfaces. The
mobility μi−v/E is the velocity of the ion in an applied electric
field E. The Rct at the anode and the cathode interface with the
electrolyte are different from one another and the charge
transport across an interface is also different between charge and
discharge, so ηch≠ηdis.
[0047] The capacity of a rechargeable battery is the amount of
charge per unit weight or volume passed between the electrodes
during a complete reaction at a constant current I=dq/dt:
Q(I)=<∫><0><Δt><Idt>=<∫><0><Q(I)>dq
(2)
An irreversible capacity loss in a charge/discharge cycle, i.e. a
Δtdis(n+1)<Δtdis(n), where (n+1) and n are cell cycle numbers,
represents a capacity fade with cycling. The coulombic efficiency
of the cell 100Δtdis(n+1)/Δtdis(n) % is a measure of the cycle
life before a rechargeable battery capacity fades to 80% of its
original capacity.
[0048] The energy density of a rechargeable battery is
ΔE=<∫><0><Δt><IVdt=><∫><0><Q(I)><V(q)dq>=<V(q)>Q(I)
(3).
where Q(I) is the capacity at a current I defined by equation (2).
[0049] For a given chemical reaction between the two electrodes of
a rechargeable electrochemical cell, a small Rel requires a thin
electrolyte with a sufficient density ni of mobile working ions
carrying a charge qi with a high mobility μi. The electronic
conductivity of a highly conductive metal is orders of magnitude
greater than any electrolyte ionic conductivity σi=niqiμi, so
rechargeable batteries are typically fabricated with a thin
electrolyte between electronically conducting electrodes that have
a large area, but the electrodes need not-have a high electronic
conductivity so long as they are not too thick and make electronic
contact to a large-area, metallic current collector.
[0050] The Rct can be made small across a solid/liquid interface,
but it is increased where a mismatch between the μA or of a solid
electrode and the LUMO or HOMO of a liquid electrolyte requires
formation of a passivating solid-electrolyte-interphase (SEI)
layer that must allow transfer of the working ion across it also.
For gaseous reactants at a solid-electrolyte surface, Rct may be
low if it is accompanied by a high catalytic activity for the
dissociation of the gas and its chemisorption into the electrolyte
or the extraction of the gas from the electrolyte. A low Rct
across a solid/solid interface is also critical. Even at an
alkali-metal anode where plating only changes the electrode
dimension perpendicular to the interface, a soft polymer interface
layer that is chemically stable on contact with the two solids may
be useful to maintain a long cycle life. If the electrode includes
small particles into which the working ion is inserted, displaces
an atom, or forms an alloy, the particle changes volume. This
volume change normally prevents the solid/solid interface from
being maintained during cycling. This problem occurs even if the
solid electrolyte is made into a paste or a melt during
fabrication to wet all the surfaces of the electrode particles.
This problem limits the battery capacity and cycle life of
previous all-solid-state batteries. However, realization of
reversible plating of an alkali metal across the solid/solid
alkali-metal/-glass electrolyte interface allows optimization of
the cell voltage for a given cathode and eliminates losses
associated with an anode SEI layer. Moreover, a solid electrolyte
blocks soluble species of a liquid redox-molecule flow-through
cathode or soluble intermediates of a sulfur cathode from reaching
the anode. However, traditional solid electrolytes, whether
glassy, amorphous, or crystalline, do not have the ionic
conductivity needed to allow their use at ambient temperature
unless they are so thin that they need to be supported by a porous
substrate or sandwiched between polymer-electrolyte membranes, and
the early report of a glass formed from a crystalline lithium
conductor did not demonstrate why it could be dry or what ionic
species was the dominant conductor. Moreover, it would be
impossible to plate an alkali metal on a copper current collector
across the solid/solid interface in the presence of liquid water
in the electrolyte.
[0051] Since the water-solvated glass/amorphous solids obtained in
this disclosure have a LUMO>EF(Li) and are stable in organic
liquid, ionic liquid, and/or polymer electrolytes, they may be
used with a liquid catholyte and/or polymer located between the
solid electrolyte and the cathode and/or with a passivating
solid-electrolyte interphase (SEI) layer and/or polymer between
the anode and the solid electrolyte. The dry water-solvated
glass/amorphous electrolytes of this disclosure open up the
possibility of using rechargeable batteries with a variety of
cathodes: conventional reversible insertion-compound solid
cathodes, redox flow-through liquid cathodes, gaseous air
cathodes, and solid sulfur cathodes. The use of a solid
lithium-ion (Li<+>) or sodium-ion (Na<+>) electrolyte
also allows a choice of a variety of electrochemical cells,
including fuel cells, electrolysis cells, and capacitor cells as
well as rechargeable battery cells.
[0052] The water-solvated glass/amorphous solid proton
electrolytes formed by exposing crystalline BaKPO4 to water vapor
can replace the NAFION membrane in an ambient temperature fuel
cell.
[0053] Rechargeable batteries containing a water-solvated
glass/amorphous solid electrolyte described herein can provide a
safe, low-cost stationary battery capable of storing a large
amount of electrical energy for feeding the grid or charging the
battery or capacitor of an electric vehicle since the temperature
range of operation of a stationary battery can be kept small
through all seasons at little cost. The small activation energy
for alkali-ion transport in the electrolyte can also make feasible
an electric vehicle powered by a portable rechargeable battery
that operates in a wide range of ambient temperatures.
Dielectrics
[0054] The water-solvated glass/amorphous solids described herein
provide huge dielectric constants that can be used in capacitors
or other devices where there is no ionic transport across the
solid/solid interface of a metallic electrode and the solid
electrolyte. The mobile ions move to the interfaces to create an
electric-double-layer capacitor and the electric dipoles in the
solid are free to rotate to add their dipole moment to the
dielectric constants. The temperature dependence of the dielectric
constants are the same as or similar to those shown in FIG. 3.
[0055] Capacitors, like batteries, store electrical energy; but
unlike a rechargeable battery or a reversible fuel cell, the
energy is stored as the electrostatic energy between electrons or
electron holes in the metallic plates of a capacitor and dipoles
or mobile ions in a solid electrolyte that separates the two
metallic plates. In a double-layer electrochemical capacitor,
mobile cations in the electrolyte attract electrons to one plate
and mobile and/or static anions attract electron holes to the
opposing plate. The mobile ions of the electrolyte are trapped by
the electrons or electron holes in the metallic plates as long as
the charging external circuit is opened, preventing the electrons
and electron holes created by charging from recombining. However,
on closing the electronic circuit, the electrons recombine
quickly, thereby releasing ion flow and dipole rotation in the
electrolyte dielectric. FIG. 5 illustrates the charge/discharge
cycling of a capacitor formed by sandwiching a thick
water-solvated glass/amorphous solid between two aluminum plates.
In the absence of carbon, the thin aluminum oxide (Al2O3) layer on
the surface of the aluminum plates blocks charge transfer across
the solid/solid interface to up to a 10 V charge. On discharge,
there are three regions versus time, one within a second that was
too fast to be recorded, one over one to three seconds that was
slow enough to be recorded with the apparatus used, and a slow
third that lasts for several minutes. The fastest presumably
reflects electron transport between trapped electrons in the anode
and electron holes in the cathodes, the intermediate discharge the
movement of cations away from the interfaces resulting from the
loss of trapped electron charge, and the slow discharge any
reorientation or diffusion of the electric dipoles.
Ionic Conductors
[0056] Electronic conduction controls electronic devices. However,
nature uses ionic conduction and redox energies to accomplish many
things. The water-solvated glass/amorphous solids of the present
disclosure may be used in devices, methods, and systems that
utilize both ionic and electronic conduction. For instance, the
trapping of electrons and/or electron holes at metal/electrolyte
interfaces may be used in an electronic memory or switch.
Exploration of the wedding of electrochemistry and electronic
devices remains a relatively unexplored domain.
[0057] According to a first embodiment, A, the disclosure provides
a method of forming a dried, water-solvated glass/amorphous solid.
The method includes transforming a crystalline, sodium ion
(Na<+>) or lithium-ion (Li<+>) electronic insulator or
its constituent precursors comprising at least one Na<+ >or
Li<+ >bonded to oxygen (O), hydroxide (OH), and/or to at
least one halide into a water-solvated glass/amorphous Na<+
>or Li<+ >ion-conducting solid by adding water in an
amount less than or equal to the water solvation limit of the
glass/amorphous solid.
[0058] In further embodiments, which may be combined with
embodiment A and with one another unless clearly mutually
exclusive, i) the method further includes adding a glass-forming
oxide, sulfide, or hydroxide and heating to expel volatile
constituents; ii) the crystalline, electronic insulator or its
constituent precursors include a material with the general formula
A3-xHxOX, wherein 0≦x≦1, A is the at least one alkali metal, and X
is the at least one halide; iii) the crystalline, electronic
insulator or its constituent precursors includes a glass-forming
additive comprising at least one of an oxide, a hydroxide, and/or
a sulfide; iv) the glass-forming additive includes at least one of
Ba(OH)2, Sr(OH)2, Ca(OH)2, Mg(OH)2, Al(OH)3, or BaO, SrO, CaO,
MgO, Al , B2O3, Al2O3, SiO2, S and/or Li2S; v) the additive
includes at least two of an oxide, a hydroxide, and/or a sulfide;
vi) the additive includes at least two of Ba(OH)2, Sr(OH)2,
Ca(OH)2, Mg(OH)2, Al(OH)3, or BaO, SrO, CaO, MgO, Al , B2O3,
Al2O3, SiO2, S and/or Li2S; vii) the dried, water-solvated
glass/amorphous solid includes less than 2 mole percent of the
glass-forming additive; viii) the additive adjusts the glass
transition temperature Tg of the water-solvated glass/amorphous
solid; ix) the at least one halide includes chlorine (Cl), bromine
(Br) and/or iodine (I); x) at least a portion of the at least one
halide exits the water-solvated glass/amorphous solid as a
hydrogen halide gas; and xi) the hydroxide reacts to form H2O that
exits the water-solvated glass/amorphous solid as gaseous H2O.
[0059] According to a second embodiment, B, the disclosure
provides a method of forming an H<+>-conductive
water-solvated electrolyte. The method includes transforming a
crystalline material comprising at least one alkali and/or
alkaline-earth cation bonded to at least one acidic polyanion into
a glass/amorphous solid by adding water in an amount less than or
equal to its solvation limit in the crystalline material such that
water dissociates into hydroixide (OH)<− >anions that
coordinate to the cations to form polyanions and the water also
dissociates into protons (H<+>) that are mobile in a
framework of an acidic oxide and the polyanions.
[0060] According to a third embodiment, C, the disclosure provides
a method of forming a water-solvated glass/amorphous solid. The
method includes transforming a crystalline electronic insulator
comprising at least one acidic polyanion and at least one cation
into a water-solvated glass/amorphous proton
(H<+>)-conducting solid by adding water in an amount less
than or equal to the water solvation limit of the crystalline
electronic insulator
[0061] In further embodiments, which may be combined with
embodiments B or C, and with one another unless clearly mutually
exclusive: i) wherein the acidic polyanion includes (SO4)<2−
>and/or (PO4)<3− >and/or (SiO4)<4− >polyanion; ii)
the at least one cation is stabilized in the form of at least one
stable hydroxide polyanion; iii) the at least one cation includes
a barium (Ba<2+>) ion, apotassium (K<+>) ion, a
rubidium (Rb<+>) ion, and/or a cesium (Cs<+>) ion; iv)
the stable hydroxide polyanion includes
(Ba(OH)x)<2-x>,(K(OH)x)<1-x>, Rb(OH)x)<1-x
>and/or (Cs(OH)x)<1-x>.
[0062] According to a fourth embodiment, D, the diclosure provides
a water-solvated glass/amorphous solid formed from the method of
any of the above embodiments. The disclosure further provides, in
additional embodiments, electrolytes and dielectrics including
this water-solvated glass/amorphous solid
[0063] According to a fifth embodiment, E, the disclousre provides
a paste or slurry including the dried water-solvated
glass/amorphous solid of embodiment D, wherein the paste or slurry
includes particles of the water-solvated glass/amorphous solid in
an organic liquid, an ionic liquid, and/or a polymer. According to
a further embodiment, the paste or slurry may be applied to a
large surface area by painting, doctor-blading, vapor deposition,
or printing.
[0064] Accoridng to a sixth embodiment, F, the disclosure provides
a method of forming an electrolyte or dielectric by applying the
paste or slurry of embodiment E to a surface. In further
embodimetns, the the organic liquid, ionic liquid, and/or polymer
may be allowed to evaporate totally or in part, leaving an
electrolyte or dielectric, or the organic liquid, ionic liquid,
and/or polymer may not be allowed to evaporate.
[0065] According to a seventh embodiment, G, the disclosure
provides a battery including a material as described above. The
battery may also inclde a liquid electrolyte, a polymer
electrolyte, or a mixture thereof, wherein the liquid or polymer
electrolyte contacts at least one electrode in the battery.
[0066] According to an eighth embodiment, H, the disclosure
provides a cell for storing electrical energy including a faradaic
and a non-faradaic component including an electrolyte material as
described above.
[0067] According to a ninth embodiment, I, the disclosure provides
a capacitor including a material as described above. The capacitor
may include two electrodes formed from the same metal or metal
alloy, or it may include two electrodes formed from two different
metals or metal alloys having two different Fermi energies.
[0068] According to a tenth embodiment, J, the disclosure provides
a fuel cell including a material as described above. The fuel cell
may be reversible.
[0069] According to an eleventh embodiment, K, the disclosure
provides an electrolysis cell including an electrolyte or
separator including a material as described above. The
electrolysis cell may produce hydrogen gas (H2) from water.
[0070] According to a twelfth embodiment, L. the disclosure
provides an electrochemical device including a reversible fuel
cell of embodiment J and a chemical storage bed.
[0071] Accordign to a thirteenth embodiment, M, the disclosure
provides an electronic device including including a material as
described above. According to further embodiments, which may be
combined with one another: i) the electronic device includes a
memory, a transistor, a switch, or a sensor including a material
as described above; ii) the electronic device uses a piezoelectric
effect of a material as described above; iii) the electronic
device uses a pyroelectric effect of a material as described
above.
[0072] Accordign to a fourteenth embodiment, N, the disclosure
provides a device that transforms heat into electric power at a
fixed temperature using a material as described above
[0073] Although only exemplary embodiments of the disclosure are
specifically described above, it will be appreciated that
modifications and variations of these examples are possible
without departing from the spirit and intended scope of the
disclosure. For instance, numeric values expressed herein will be
understood to include minor variations and thus embodiments
“about” or “approximately” the expressed numeric value unless
context, such as reporting as experimental data, makes clear that
the number is intended to be a precise amount. In addition, the
water-solvated glass/amorphous solids may be used in batteries and
capacitors and other electrical or electrochemical devices having
components and properties that are otherwise known and that are
described in the background.
US2017005327
CATHODE ADDITIVE FOR RECHARGEABLE SODIUM BATTERIES
Inventor(s): GOODENOUGH JOHN, et al.Also published as:
WO2017004121 (A1)
The present disclosure relates to a cathode additive for a
rechargeable sodium battery, to mixtures of the additive and a
cathode active material, to cathodes containing the additive, to
electrochemical cells with cathodes containing the additive, and
to rechargeable batteries with cathodes containing the additive.