
rexresearch.com
Ralph MOODY, Jr.
Back Pressure Turbocharger
Ralph Moody, Jr.got 84 mpg from a Ford
Capri modified with a turbocharged back-pressure regulator on
4 cylinder Perkins diesel engine.
http://www.abovetopsecret.com/forum/thread945991/pg1
Ralph Moody and the Moodymobile
...So I was at work, and working in a hospital, we began
discussing healthcare and the financial problems that are
resulting from the low reimbursements which are directly impacting
patient care. The conversation then progressed to other ways the
government is screwing people, and ended with this ...
My coworker has an uncle whom I had not heard of, Ralph Moody. She
said she forgets what he did, but he created an 80+ mpg car 30
years ago, that garnered offer from foreign interests in excess of
20 million. She said her uncle was very patriotic, and turned them
down wanting to sell the technology to an American car company
perhaps, but not to a foreign group. This car, which did not start
out as a low MPG project, utilized a carburetor they created to
achieve these outstanding results. The federal government showed a
lot of interest in the car, so much that they confiscated it. My
coworker said the car still sits in a storage area in North
Carolina and that her family is allowed to use it, but the
property can not be passed down, it no longer belongs to them. I
am not one to believe in these magic carburetors, I would love to
know more about this particular car...
http://www.caranddriver.com/columns/al-gore-wasnt-the-only-guy-flogging-an-80-mpg-car
Al Gore Wasn't the Only Guy Flogging an
80-mpg Car
by PATRICK BEDARD
"What happened to this?" asks reader Pete Kontes about a 1979 AP
wire story clipped from his local paper, the Post-Register, in
Idaho Falls, Idaho.
The headline screams, "Former racer could have energy crisis
killer."
Readers of a certain age will recognize 1979 as the time between
Energy Crisis One and Energy Crisis Two, a grim era of fuel
shortages and lines at gas pumps following the OPEC embargo of
1973-74.
The "racer" here was Ralph Moody, most famous as the mechanical
wizard who had teamed up with super salesman John Holman to form
Holman-Moody, which went through NASCAR in the '60s like Patton
had gone through Africa two decades before.
According to the old clipping: "In tests recently at Daytona Beach
Community College, Moody's 1979 Mercury Capri test car got an
astounding 84 miles to a gallon. The skeptical test supervisor,
Bill Gordon, who has supervised Environmental Protection Agency
fuel economy tests before, couldn't believe it. 'It is the car of
the century,' Gordon said enthusiastically after the test."
The article's description of the engine sounds confused. Moody is
quoted as saying, "We took a four-cylinder Perkins block,
converted it to diesel fuel, turbocharged it, and built a special
clutch, transmission, and rear-end setup."
Other sources simply say it was a Perkins diesel, which sounds
more likely.
Those years of iffy fuel supply had the car market in a panic,
with frugal Japanese sedans selling far above sticker price and
Detroit still caught in the Cutlass Supreme era of chromey
two-door hardtop coupes about the size and weight of today's SUVs
but with no space in the back seat. American Motors had already
responded by dragging its compact Hornet sedan out behind the barn
and whacking off most of the useful parts—all of the trunk and a
major section of the back seat—to create the worthless Gremlin.
Motorists wanted miles per gallon, and they wanted it instantly.
But elephants can't zig. Detroit factories were mostly tooled for
big iron. The industry seemed unresponsive, and the media had the
persistent notion that some guy somewhere in a one-car garage,
armed with only a screwdriver and a Crescent wrench, could
outengineer General Motors. The papers were filled with breathless
stories like the one of Moody's Capri.
"We just got flooded" with inventors, Howard Padgham remembered
recently. He's retired now after years heading Chrysler's
powertrain engineering. But neither he nor I remembered Moody's
car.
I spent a week in Moody's shop back in 1974 doing field surgery on
this magazine's full-race Pinto after an inglorious debut at
Talladega. Within hours of leaving, we qualified on the pole for
the Charlotte race and later won it with a big lead. I'm sure the
other competitors thought Moody had uncorked a few speed secrets
for me.
If he had sure-fire recipes, he didn't share them. He seemed a
sensible guy, careful about the details as he understood them. He
left the big talk to others.
The rumor had been floating around about a Moody mileage maker for
a few months before the Capri appeared, something on the order of
60 to 70 mpg. About these numbers, he said later, "They were a
little conservative—on purpose. If you come right out saying
you've got a car that'll get 80 to 90 miles per gallon, people
will think you're some kind of jerk."
Yes, that sounds like the Ralph Moody I knew. He was no Smokey
Yunick. But we'll come to the Smoke's mileage maker in a bit.
Padgham confirms another detail of the times. Most of the
inventors who came forward with magic miles-per-gallon numbers
"were normal people, not charlatans." They set up their tests at
constant speeds, on level roads, with their engines fully warmed
up. In that driving, even the relatively primitive engines of the
time went far on a gallon.
Moody died last summer, so we can't ask him about the Capri, but I
did find it listed in Government Involvement in Suppressed
Inventions, by Pea Research (iaesr.homestead.com). The lengthy
compendium confirms that carburetors were favorite breakthroughs
for Uncle Sam's squelching, along with a reassuring number of
flying saucers. My thanks to Pea Research for answering reader
Kontes's question more eloquently than I could have.
Before we leave the topic of government suppression, however,
let's put Moody's achievement in perspective: "They" also got to a
"highly modified 1959 Opel" credited with 376.59 mpg.
I don't doubt Padgham when he says most of the inventors weren't
charlatans, but it wasn't always easy to tell the difference.
Early in 1984 I went down to "The Best Damn Garage in Town," town
being Daytona Beach, Florida, along with Don Sherman, C/D
technical director at that time, for a look at Smokey Yunick's
"phase-one adiabatic hot-vapor" engine. It was a 2.2-liter
Chrysler four promising 0 to 60 in six seconds and 50 mpg.
Sherman and I are both engineers by training and wary of those who
claim to have found an end run around the laws of thermodynamics.
Yunick had been doing his rulebook double-shuffle against NASCAR's
canniest tech inspectors for decades before we arrived. We didn't
expect a PowerPoint presentation. What we got was the full wizard
act, a sinewy guy in the white coveralls you see "before" in the
detergent commercials. Even at breakfast I don't remember that he
removed his bad-cowboy black hat. It was oil-soaked at the band
and permanently embedded with aluminum chips from his machine
shop.
He was 60 then. I think the man and his act were already
inseparable. If you fell for the act, maybe you wouldn't notice
the fundamental outrageousness of his claims. He had a
high-compression engine with a turbocharger. Very risky with the
crude controls of mixture and timing of those days. The usual
technique then and now is to add an intercooler to the intake
stream. Yunick had an interheater instead. Yes, retaining heat
helps efficiency, at least theoretically, but you might just as
well put a .357 Magnum to the cylinder head.
We went out for a test drive. The car pulled like hell. It pinged
like hell, too, and busted a piston early in the acceleration
runs.
Yunick stuck to his story. We didn't buy it. Later I heard that he
had sold his "hot vapor" patents to some big company, along with a
multi-year consulting contract that would keep the cash flowing
into his pockets. For the honest inventors and for the charlatans
alike, that was always the touchdown.
Was he on to something we simply couldn't comprehend? Let's just
say, two decades and many mpg regulations later, I don't see any
"hot vapor" engines.
http://people.com/archive/ralph-moody-may-travel-the-road-to-riches-in-a-diesel-car-that-gets-84-miles-per-gallon-vol-11-no-20/
Ralph Moody May Travel the Road to Riches
in a Diesel Car That Gets 84 Miles Per Gallon
By Sandra Hinson
About a year ago Ralph Moody, a legendary stock car driver and
mechanic, was thinking about retiring. “All I wanted to do,” he
says, “was a little bit of nothing.” Today Moody has postponed the
rocking chair in favor of rocking the auto industry. He and a pal
have designed a car with an engine that gets up to 84 miles to a
gallon of diesel fuel. The Moodymobile, as it’s called, came out
of the Oak Hill, Fla. auto shop of Mike Shetley, whom Moody knew
from their days together on the Ford racing team. Shetley had
summoned his old boss from his Charlotte, N.C. home to help with a
complicated chassis for a Thunderbird replica. Moody, 60, solved
the problem in a week, and soon the men were talking about
building a sports car with a diesel engine. “The idea wasn’t high
mileage in the beginning,” says Shetley, 36. “We wanted a
nice-driving car for the guy who couldn’t afford luxury.”
What they did was modify, inside and out, a four-cylinder Perkins
diesel engine (like those used in motorboats) and drop it into a
1979 Capri body, adding a turbocharger for extra power. (The
turbocharger reroutes hot exhaust gases that normally escape from
the tail pipe.) The Moodymobile was soon stirring interest locally
and was test-driven by Congressman Bill Chappell. He immediately
wired President Carter: “I’ve seen it, I’ve driven it and it
works.”
A fortnight ago Moody and Shetley drove the car 850 miles to
Washington, D.C. (on 11.1 gallons of fuel) and testified before
the Senate Energy Committee. The car (which is noisier than a
conventional one) faces a stiff battery of environmental tests
before it can be marketed, but Moody is confident one of the big
automakers will buy the rights to the patent. Ford, Chrysler and
General Motors have all expressed interest.
Born in Massachusetts, Moody raced midget cars as a teenager and
in 1956 joined Ford, later becoming chief mechanic. That meant
giving up competitive driving. “I could still outrun them,” he
proclaims. “I did all the test work.” Champion drivers like Mario
Andretti, A.J. Foyt, Cale Yarborough and Bobby Allison have won
with Moody-groomed Fords. He also designed much of the safety
equipment now standard in stock cars.
When Ford stopped racing in 1971, Moody opened a small “speed
shop” in Charlotte (a city he, his wife Mitzi, 53, son Ralph III
and daughter Ann have lived in for 23 years). He began selling
race cars to drivers and, as a sideline, refined a gasoline engine
that gets up to 65 miles per gallon. “We put it on the shelf,”
Moody shrugs. “Nobody needed it then.”
Shetley claims — perhaps extravagantly — that they have turned
down $100 million from Arab interests to buy rights to the
Moodymobile, which has cost them $20,000 to develop. Meanwhile,
the designers are filing away in cardboard boxes the names of
private citizens eager to place orders. Possibly 2,000 of the cars
will be available late this year for about $7,000 apiece. And for
the time being, Ralph Moody has put off retirement. “In the old
days on the racing circuit, I worked day and night,” he smiles.
“Now it’s only 20 hours a day.”
Popular Mechanics ( August 1979 )
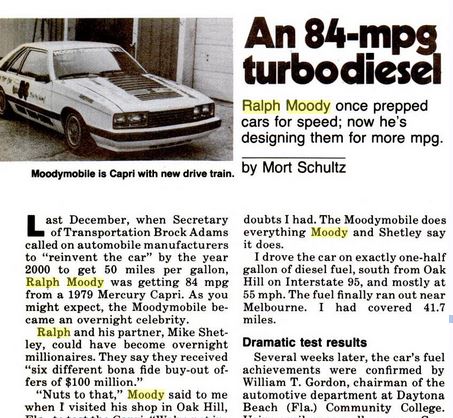

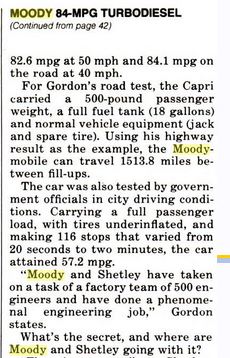
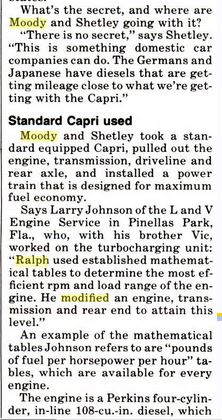
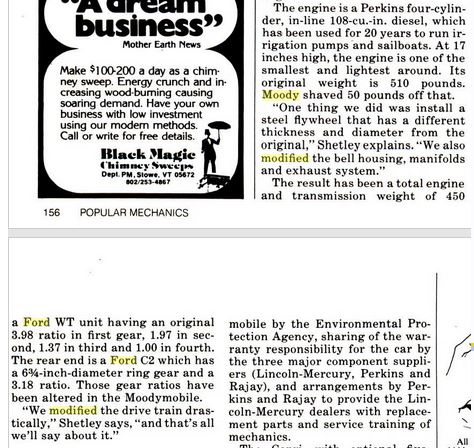
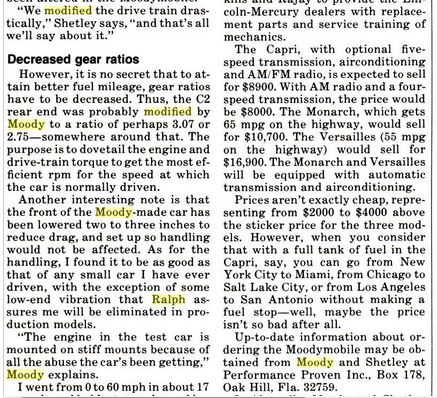
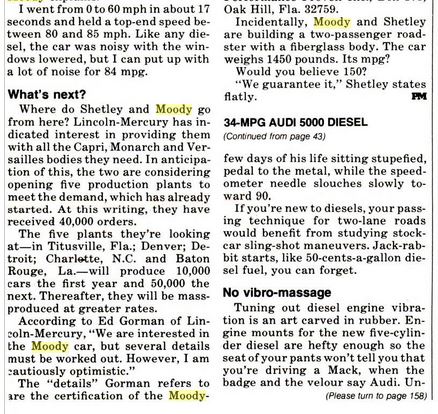
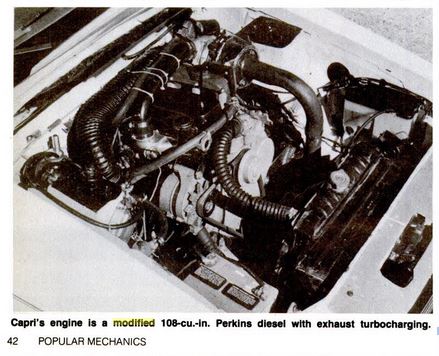
"Osculate my tailpipe, Detroit !"

Ralph Moody Jr.
WO9216725
APPARATUS REGULATING EXHAUST FLOW TO INCREASE BACK PRESSURE
IN AN INTERNAL COMBUSTION ENGINE
Inventor: MOODY RALPH A JR
The internal combustion engine (10) includes a fuel delivery
system (200) and an exhaust system having an apparatus (44) for
regulating exhaust flow to increase engine back pressure. In
response to regulated exhaust flow and increased engine back
pressure, the fuel delivery system (200) is controlled to decrease
fuel flow resulting in increased fuel efficiency of the engine and
decreased exhaust emissions. A forced air induction system such as
a turbocharger (80) or supercharger may also be used in conjuction
with the engine (10). In preferred embodiments, the exhaust flow
regulating apparatus (44) is either a fixed cross-sectional area
orifice or a variable cross-sectional area orifice.
Background of the Invention
Internal combustion engines are widely used to provide power for
vehicles and machinery, and therefore, it is desirable to design
these engines so that fuel consumption and emissions are reduced.
Air flow through conventional diesel internal combustion engines
is not controlled and exhaust flow is generally increased by
designing these engines with reduced exhaust restrictions. Air
flow through conventional gasoline engines is controlled by
restrictions in the induction side which create lower than wide
open throttle combustion pressures resulting in combustion
efficiency losses under normal operation. Prior art teaches that
internal combustion engine efficiencies are improved by reducing
exhaust system restrictions.In a Society of Automotive Engineers
article titled "The Influence of the Exhaust Back Pressure of a
Piston Engine on Air Consumption, Performance, and Emissions",
January 8-12, 1973, the authors showed that engine air consumption
responds to variation of the ratio of absolute exhaust back
pressure to absolute inlet manifold pressure with a strong
dependence on engine speed, and that exhaust back pressure affects
performance and lowers some exhaust emissions. The authors,
however, did not investigate how fuel efficiency is affected when
exhaust flow is regulated to increase engine back pressure while
at the same time reducing fuel flow.
Summary of the Invention
The internal combustion engine of the invention includes a fuel
delivery system and an exhaust system having an apparatus for
regulating exhaust flow through the engine to increase engine back
pressure. In response to regulated exhaust flow and increased
engine back pressure, the fuel delivery system is controlled to
decrease fuel flow resulting in increased fuel efficiency. Reduced
exhaust emissions also result. A forced air induction system such
as a turbocharger or supercharger may also be used in conjunction
with the engine.
In general, the apparatus for controlling exhaust flow and
increasing engine back pressure is any restriction positioned
within the exhaust system of either a diesel or gasoline (spark
ignition) internal combustion engine. In one embodiment the
apparatus is a venturi system having a large opening tapering to a
smaller opening that allows exhaust to flow from the large opening
through the smaller opening. Alternatively, the exhaust flows
through a venturi system having an opening formed by a fixed side
and a movable side adjustable at various engine operating
parameters by an actuating system to establish a desired increase
in back pressure.
The internal combustion engine of the invention directly
contradicts the prior art in that back pressure is increased
resulting in increased fuel efficiency. The advantages of the
internal combustion engine of the invention are that fuel
efficiency is increased because induction side losses of the gas
engine can be reduced, the dynamic combustion pressures of both
diesel and gas engines are increased, exhaust flow in both diesel
and gas engines is controlled, and when utilized in conjunction
with a forced air induction system such as a turbocharger or
supercharger, the invention eliminates the need for and efficiency
losses of a dump valve or wastegate valve that is normally
required for forced air induction system overpressure protection.
Brief Description of the Drawings
Fig. 1 is a perspective view of one embodiment of the
internal combustion engine of the invention;
Fig. 2 is a cross-sectional view of apparatus for
regulating exhaust flow to increase engine back pressure having
a large opening tapering to a smaller opening;
Fig. 3 is a cross-sectional view of apparatus having a
variable orifice for adjustably regulating exhaust flow to
increase engine back pressure;
Fig. 4 is a cross-sectional view taken along line 4-4 of
the Fig. 3 apparatus;
Fig. 5 is a perspective view of the internal combustion
engine of the invention showing a turbocharger connected to the
exhaust system;
Fig. 6 is a side view of the turbocharger shown in Fig. 5;
Fig. 7 is a front view with parts broken away from the
turbocharger shown in Fig. 5;
Fig. 8 is an alternate perspective view showing a
controllable fuel delivery system;
Fig. 9 is a graph showing intake manifold boost pressure as
a function of RPM;
Fig. 10 is a graph showing exhaust manifold back pressure
as a function of RPM; and
Fig. 11 is a graph showing the ratio of boost pressure to
back pressure at maximum acceleration conditions expressed in
gauge pressures as a function of RPM.
Description of the Preferred Embodiments
As shown in Fig. 1, an internal combustion engine 10 includes a
cylinder head 12, intake manifold 14, exhaust manifold 16 and a
controllable fuel delivery system 200 as shown in Fig. 8. The
exhaust manifold 16 includes a pair of hollow spaced legs 18 and
20 connected to the cylinder head 12 by plates 22 and fasteners
24. Alternatively, plates 22 can be welded to the cylinder head 12
or be cast integrally with the cylinder head 12 with the prime
consideration being that whatever mode of connection is used the
connection should be substantiaffily airtight. The free ends of
legs 18 and 20 merge at 26 and 28 respectively, preferably in a
smooth curvilinear manner, into a conduit passageway 30 disposed
parallel to and spaced from the cylinder head 12.
The free end of the conduit 30 terminates at an exhaust section
32, this section preferably being a smooth curved section, to
which a mounting flange 34 is secured. The flange 34 removably
supports an exhaust pipe 36 having an inlet 38 terminating in a
flange 40 complementally fastened to the flange 34 by nuts and
bolts 42 or similar fasteners.
The controllable fuel delivery system 200 as shown in Fig. 8
comprises a fuel tank 137, fuel line 143, transfer fuel pump 142,
fuel line 141, fuel filter 140, fuel line 139, fuel flow control
lever 160, conventional rotary style fuel injection pump -133,
injector lines 135, fuel injectors 134, return fuel line 138 from
the fuel injectors 134, and return fuel line 136 from the fuel
injection pump 133. Fuel flows from the fuel tank 137, to the
transfer fuel pump 142, through the filter 140 to the fuel
injection pump 133. Rate of fuel flow from the fuel injection pump
133, via lines 135 to the fuel injectors 134, is controlled by the
fuel flow control lever 160. Residual fuel that is not injected
into the engine is then returned to the fuel tank 137 via the
return lines 136 and 138. The purpose for the return lines 136 and
138 is twofold. First, the return lines 136 and 138 allow for pump
and injector cooling, especially under low load or idling
conditions, and second, they allow for venting of unwanted gases
that may accumulate in the system. The fuel flow spray pattern of
the controllable fuel delivery system 200 may be a cone shaped
fuel flow spray pattern.
It has been found that by regulating the exhaust flow exiting the
engine to increase engine back pressure, air flow entering the
engine can be controlled, and engine efficiency will be
significantly improved when fuel flow is also reduced. Exhaust
emissions will be significantly reduced as compared with
conventional diesel and gas internal combustion engines.
As shown in Fig. 2, this result is obtained by placing apparatus
for controlling exhaust flow to increase engine back pressure
within the exhaust system. A venturi system 44 is placed between
exhaust section 32 of the conduit passageway 30 and the inlet 38
of the exhaust pipe 36. The venturi system 44, in its simplest
form, comprises a funnel-like member 46 having a large opening 48
tapering to a smaller opening 50. A flange 52 disposed about the
opening 48 is removably secured between exhaust section flange 34
and inlet flange 40 by fasteners 42 for keeping the venturi system
44 in position. The venturi system 44 may also be formed as an
integral part of exhaust section 32 of conduit 30 or of the inlet
38 of the exhaust pipe 36.
Exhaust flows from the large opening 48 through the smaller
opening 50, thus regulating exhaust flow to increase engine back
pressure. Operation of the internal combustion engine 10
incorporating the fixed venturi system 44 of Fig. 2 is identical
to the operation of existing internal combustion engines commonly
used in vehicles. The fixed venturi system 44 can also be
incorporated into engines, such as industrial engines, operating
under constant loads.
Alternatively, as shown in Figs. 3 and 4, a venturi system 44 is
constructed as a variable cross-sectional area venturi system
which can be utilized in engines, such as automobile engines,
operating under a range of dynamic loads. Exhaust section 32 of
conduit 30, shown in Fig. 1, transitions from a round to
rectangular shape at section 56 terminating at rectangular section
58 having a rectangular flange 60. The variable venturi system 44
is positioned at the rectangular inlet 62 of exhaust pipe 64, such
rectangular inlet 62 having a rectangular inlet flange 66
connected to rectangular flange 60 by nuts and bolts 68.
The variable venturi system 44 comprises a fixed converging side
70, and a moveable side 72, which rotates with a shaft 74 movable
by an arm 76 having a hole 78 for connection to an actuating
device (not shown). In addition to increasing engine back pressure
and reducing fuel flow, the variable venturi system 44 of the
invention may be used as the engine's main control, thus
eliminating the induction side throttling device currently
required for operation of non-diesel engines.
Rudimentary operation of the internal combustion engine 10
incorporating the variable venturi system 44 of Figs. 3 and 4 may
be accomplished by connecting the throttle pedal (not shown) to
the fuel flow control lever 160 of Fig. 8 and the arm 76 of Fig. 3
by cam linkages. Actuation of the throttle pedal will change the
position of the fuel flow control lever 160 and cause the arm 76
to vary the position of the movable side 72 of the venturi system
44.
In another embodiment, operation of the internal combustion engine
10 incorporating the variable venturi system 44 is effectuated by
a microprocessor (not shown) that receives information from engine
sensors (not shown) that measure various operating parameters. The
microprocessor analyses the received information and sends optimum
position output information to the fuel flow control lever 160 and
arm 76 which, in turn, positions the movable side 72 of the
venturi system 44. Examples of sensors that may be incorporated
into the internal combustion engine 10 of the invention are
sensors that measure oxygen concentration, coolant temperature,
manifold air pressure, vehicle speed, throttle position, engine
RPM, mass air flow, detonation (anti-knock), exhaust temperature,
exhaust manifold pressure and fuel flow.The throttle pedal,
connected to an input transducer (not shown), in effect, acts as
the throttle position sensor. When a forced induction system such
as a turbocharger is used in conjunction with the engine, as
discussed below, additional sensors that measure boost pressure
and turbocharger RPM may also be used for optimization of the
positions of the fuel flow control lever 160 and the movable
venturi system 44.
During engine idle, the variable venturi system 44 and fuel flow
control lever 160 are positioned at a predetermined minimum
setting. The fuel flow, controlled by the fuel flow lever 160, is
continuously and automatically adjusted to maintain proper fuel
mixture based on engine speed, mass air flow, and oxygen
concentration sensor input. The position of arm 76, as shown in
Fig. 3, is continuously and automatically adjusted for idle speed
control. Because engine load may vary at idle due to accessory
demands, the movable side 72 is constantly repositioned to
maintain minimum idle speed.
Upon desired acceleration, the throttle position sensor delivers
an increasing voltage to the microprocessor which, in turn,
increases the opening of the variable venturi system 44. Based on
input from other operating sensors, the fuel flow control lever
160 is adjusted to regulate fuel flow resulting in an optimum air
to fuel ratio. If maximum engine speed is achieved inadvertently,
the microprocessor can reduce engine speed by reducing fuel flow
and/or air flow by repositioning the fuel flow control lever 160
and/or the movable side 72 of the venturi system 44. In addition,
if the engine is equipped with a turbocharger, the manifold air
pressure sensor will supply input to the microprocessor causing
the microprocessor, upon approach of maximum inlet manifold boost
pressure, to begin closing the variable venturi system 44.As this
action occurs, the mass air flow and the fuel flow will be reduced
to maintain air to fuel ratios within an acceptable range.
Oxygen concentration sensors can also be used in conjunction with
mass air flow sensors for maintaining air to fuel ratios. If the
boost pressure increases beyond a desired maximum, the
microprocessor will respond by initially causing the fuel flow
control lever 160 and the movable side 72 to close until an
acceptable air flow and manifold pressure is achieved. The engine
will then return to the desired boost operating mode and optimum
air flow to fuel flow will be retched by the gradual opening of
the venturi 44 which is limited by the manifold air pressure
sensor. When the opening of the venturi 44 is limited, the
microprocessor will also limit the opening of the fuel flow
control lever 160 to maintain acceptable fuel to air ratios while
controlling maximum boost pressure.It is noted that the fixed
venturi system 44 of Fig. 2 cannot exceed a maximum boost pressure
because the smaller opening 50 is designed to control the maximum
boost pressure.
At maximum deceleration, fuel flow is completely shut off by
closing the fuel flow control lever 160, and the movable side 72
of the venturi system 44 is returned to a preset minimum position.
When the engine approaches idle speed, the fuel flow is turned
back on and the engine begins operating under idle conditions.
Moderate deceleration is achieved by varying the position of the
movable side 72 based on input from various operating sensors
including the throttle position sensor. Fuel flow to fuel
injectors 134, as shown in Fig. 8, is reduced proportionally as
air flows are reduced by the closing of the variable venturi
system 44.
As previously mentioned, the internal combustion engine 10 of the
invention may be used in conjunction with a forced air induction
system such as a turbocharger. Figs. 5 and 6 show a removable
turbocharger 80 attached to the exhaust section 32 of the internal
combustion engine 10 at mounting flange 34 removably supporting
the turbocharger 80. A housing 82 included in turbocharger 80
comprises a tangentially disposed conduit section 84 extending
outwardly from the housing 82 with its free end 86 terminating at
flange 88 complementally fastened to flange 34 by nuts and bolts
42 or similar fasteners.
Further details of the turbocharger 80 are illustrated in Figs. 5,
6 and 7, and include a totally enclosed generally cylindrical
outer housing 82 having an axially disposed air inlet 90 having
conduit means 91 and an axially disposed exhaust outlet 92 having
conduit means 93, preferably at opposite ends thereof. An
additional radially positioned opening 94 is provided adjacent to
air inlet 90 and communicates therewith to convey air entering
inlet 90 to the intake manifold 14 by conduit means 96 removably
secured at one end to opening 94 and at the other end 98 to an
opening 100 provided in the wall of intake manifold 14.
The housing 82 further includes rotor housing sections 102 and
104, preferably bulbous-like, directly behind the axial disposed
openings 90 and 92 and receiving rotors 106 and 108 each including
a plurality of blades 110 and 112, respectively, radially disposed
about a hub in conventional fashion. The rotors 110 and 112 are
mounted on a common shaft mounted for rotation within housing 82.
A recessed section 114 connects the rotor housing sections 102 and
104 and an oil line 116 communicating with an oil source (not
shown). The oil line 116, positioned at the top of housing 82,
discharges oil into the interior of housing 82 to lubricate the
bearings rotatably supporting the rotor shaft. An oil return line
118 positioned at the bottom of recessed section 114 returns oil
to the source.
The housing 82 is further seen to be formed of section parts to
permit ready access to the interior thereof, and to this end, the
rotor housing section 104 is formed with a flange 120 which is
removably secured to flange 122 of recessed section 114 by a
standard peripheral clamp (not shown) similar to standard
peripheral clamp 130. The other flange 126 of recessed section 114
mates with flange 128 of rotor housing section 102 and is held in
place by the standard peripheral clamp 130 having a nut and bolt
means for holding the clamp 130 in place. A gasket 132 or the
like, is used (only one being shown) to make the joints between
flanges 120, 122, 126, 128 airtight.
While the weight of the turbocharger 80 is mainly supported by
exhaust manifold 16 and its connection thereto, the conduit means
91, 93, and 96 associated therewith, also aid in the suspension of
the turbocharger as the free ends thereof are connected to other
supporting structures The free end of conduit 91 is connected to
an atmospheric opening, not shown, in the vehicle or machine body,
and the free end of the conduit 93 is connected to the exhaust
pipe of the vehicle or machine, and the air conducting conduit 96
is connected to the intake manifold 14. The conduits 91, 93, and
96 can be made of any suitable material but it preferred that
conduit 91 be made of rubber or the like of the bellows variety to
facilitate connection of the conduit 91 to component parts.The air
conducting conduit 96 is preferred to be constructed of metal due
to temperature and pressure considerations, connected at ends 100
and 94 via rubber like hose and metal clamps to facilitate removal
for inspection or repair. The exhaust conduit 93 is made of metal
as it is in contact with high exhaust temperatures.
Up to this point, the operation of the turbocharger is standard in
that the gases emanating from the exhaust manifold 16 at section
32 are directed against the blades 112 of rotor 108 thereby
imparting rotation to the rotor 108, such rotation causing rotor
106 to rotate with the blades 110 drawing atmospheric air through
conduit 91 into the rotor housing 102 from where it is discharged
through conduit 96 into the intake manifold 14 to increase intake
air pressure.
It is known that present day turbochargers are designed in such a
manner that no consideration is given to the control and use of
the air processed by the turbocharger. A dump valve or wastegate
valve is associated with known turbochargers which opens to vent
exhaust when too much exhaust is available in the turbocharger.
This defect, as is apparent, then places additional strain on the
engine in that it causes both the turbocharger and the engine to
process unwanted additional volumes of exhaust that are then
similarly discharged to the atmosphere via the dump valve or
wastegate valve which is normally located in the exhaust manifold
prior to the turbocharger. These dump valves or wastegate valves
are "pop-off" relief valves actuated by the sensing of excess
pressure at the intake of the engine.
Upon actuation, these valves vent exhaust gases to the atmosphere,
thereby preventing them from flowing through the turbocharger
which would in turn generate additional intake pressures via the
turbocharger function.
It has been found that by regulating the exhaust flow exiting the
engine, air flow entering the engine and boost pressure produced
by the turbocharger can be controlled, and the fuel efficiency of
the engine or engine-turbocharger combination will be
significantly improved. Exhaust emissions are also substantially
reduced as compared to conventional diesel and gas engines because
controlled exhaust flow and increased back pressure provide for
more complete combustion.
The apparatus for regulating exhaust flow to increase engine back
pressure as previously discussed and shown in Fig. 2 is
incorporated into the engine-turbocharger combination to increase
fuel efficiency and reduce exhaust emissions.
The first test engine-turbocharger combination was a Perkins
marine diesel engine with a displacement of 108 cubic inches
fitted into the chassis of a 1979 Mercury Capri. All sharp edges
or contours of combustion area surfaces of the engine, such as
piston top surfaces and surfaces within the cylinder head
combustion chamber and preignition chamber, were slightly rounded
to reduce the potential of concentrated "hotspots" during
operation under leaner fuel ratios. These slight engine
modifications were made to extend engine life and do not
significantly alter or improve the operation of the engine. The
turbocharger attached to the engine was a Rayjay turbocharger,
model #3881882581. Total vehicle weight was 3,300 pounds.The fixed
venturi system 44 of Fig. 2 was incorporated into the first test
engine-turbocharger combination wherein the cross-sectional area
of the large opening 48 was approximately 2.1 square inches, and
the cross-sectional area of the smaller opening 50 was
approximately 0.44 square inches resulting in an approximate 4.7:1
cross-sectional area ratio. The fixed venturi system 44 was
secured between mounting flange 34 and flange 88 as shown in Fig.
6 by nuts and bolts 42.
The first test engine-turbocharger combination having the fixed
venturi system of Fig. 2 was extensively tested.
Certified Environmental Protection Agency mileage and emissions
tests were run with the following results:
Cold start city test: 37.10 miles per gallon
3.40 grams per mile of Carbon Monoxide
0.41 grams per mile of Hydrocarbons
1.00 grams per mile Oxides of Nitrogen
Highway test: 56.23 miles per gallon
2.10 grams per mile of Carbon Monoxide
0.33 grams per mile of Hydrocarbons
0.71 grams per mile Oxides of Nitrogen
At a steady state of 55 miles per hour 63.97 miles per gallon was
achieved.
Test results, as shown by the graphs of Figs. 9 and 10, indicate
that the exhaust manifold back pressure and intake manifold boost
pressure can be significantly altered by the fixed venturi system
44. Increased back pressures on the engine show additional torque
(horsepower) gains in the lower RPM ranges on both naturally
aspirated and turbocharged engines. Horsepower gains are slightly
higher in forced air induction system applications due to the
added effect of slightly increased boost pressures at lower RPMs.
Test results, however, indicate that engine output horsepower is
not dramatically affected with back pressures exceeding 2
atmospheres, and lower RPM boost pressures can be increased while
flattening the upper RPM boost pressure curve as shown in Fig. 11.
The ratio of boost pressure to back pressure varies during the
several operational conditions including maximum acceleration,
maximum deceleration, and idle. As shown in Fig. 11, the maximum
boost pressure to back pressure ratio for the first test
engine-turbocharger combination operating at maximum acceleration
was approximately 0.27.
The variable orifice venturi system 44 of Figs. 3 and 4 may also
be incorporated into the engine-turbocharger combination by
forming the free end 86 of conduit section 84 of the turbocharger
shown in Fig. 6 into a rectangular free end 86 having a
rectangular flange 88 to complementally join with rectangular
mounting flange 60 by nuts and bolts 68 as shown in Fig. 3. As
shown in Fig. 6, the variable orifice venturi system 44 includes a
fixed side 70 integrally formed with the turbocharger free end 86,
movable side 72, and additional elements as previously discussed.
At higher engine RPM, the orifice formed by the fixed side 70 and
the movable side 72 will be larger than when operating at a lower
RPM, except in the case where boost pressure begins to exceed an
upper control limit predetermined by engine and turbocharger
parameters. When boost pressure approaches the upper control limit
the opening action of the variable orifice venturi system 44 is
retarded, and is reversed as the boost pressure meets the upper
control limit. This closing of the variable orifice venturi system
44 further increases back pressure on the engine, thereby reducing
exhaust flow, which in turn slows the turbocharger rotation
resulting in reduced boost pressure and eliminating the need for a
dump or wastegate device.